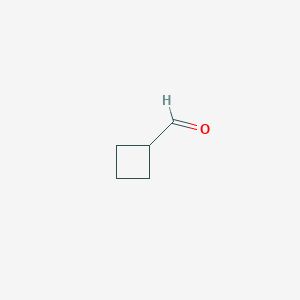
Cyclobutanecarboxaldehyde
Overview
Description
Cyclobutanecarboxaldehyde is an organic compound with the chemical formula C5H8O. It is a colorless liquid characterized by a pungent odor. This compound is known for its cyclobutane ring structure and an aldehyde functional group, making it a valuable intermediate in organic synthesis . This compound is used in the preparation of various pharmaceuticals, pesticides, fragrances, and cosmetics .
Preparation Methods
Cyclobutanecarboxaldehyde can be synthesized through several methods:
Oxidation of Cyclobutanemethanol: This method involves the oxidation of cyclobutanemethanol using reagents such as sodium hypochlorite and potassium bromide in dichloromethane.
Reduction of Cyclobutanecarboxylic Acid Chloride: This method uses lithium tri-t-butoxyaluminum hydride at low temperatures to reduce cyclobutanecarboxylic acid chloride to this compound.
Rosenmund Reduction: This method involves the reduction of cyclobutanecarboxylic acid chloride using hydrogen gas and a palladium catalyst.
Chemical Reactions Analysis
Cyclobutanecarboxaldehyde undergoes various chemical reactions, including:
Oxidation: In the presence of oxygen, this compound can be oxidized to cyclobutanecarboxylic acid.
Reduction: It can be reduced to cyclobutanemethanol using reducing agents such as sodium borohydride.
Substitution: This compound can undergo nucleophilic substitution reactions, forming various derivatives.
Common reagents used in these reactions include sodium hypochlorite, lithium tri-t-butoxyaluminum hydride, and sodium borohydride. The major products formed from these reactions are cyclobutanecarboxylic acid, cyclobutanemethanol, and various substituted derivatives .
Scientific Research Applications
Cyclobutanecarboxaldehyde has several applications in scientific research:
Chemistry: It is used as an intermediate in the synthesis of complex organic molecules.
Biology: This compound is used in the study of enzyme-catalyzed reactions and metabolic pathways.
Medicine: It serves as a precursor in the synthesis of pharmaceutical compounds.
Industry: This compound is used in the production of fragrances, pesticides, and cosmetics.
Mechanism of Action
The mechanism of action of cyclobutanecarboxaldehyde involves its reactivity due to the presence of the aldehyde functional group. This group can participate in various chemical reactions, such as nucleophilic addition and oxidation. The molecular targets and pathways involved include interactions with nucleophiles and oxidizing agents, leading to the formation of different products .
Comparison with Similar Compounds
Cyclobutanecarboxaldehyde can be compared with other similar compounds, such as:
Cyclobutanecarboxylic Acid: Unlike this compound, this compound has a carboxylic acid functional group, making it more acidic and less reactive in nucleophilic addition reactions.
Cyclobutanemethanol: This compound has a hydroxyl group instead of an aldehyde group, making it more suitable for oxidation reactions to form this compound.
Cyclobutanone: This compound has a ketone functional group, which makes it less reactive in nucleophilic addition reactions compared to this compound.
This compound is unique due to its aldehyde functional group, which provides distinct reactivity and selectivity in various chemical reactions .
Biological Activity
Cyclobutanecarboxaldehyde (CBCA), an organic compound characterized by a cyclobutane ring with an aldehyde functional group, has garnered attention in recent years for its potential biological activities. With a molecular formula of C4H6O and a molecular weight of 84.12 g/mol, CBCA's unique structure allows it to participate in various chemical reactions, making it a candidate for drug development and other applications.
CBCA can be synthesized through several methods, including the enantioselective [2 + 2] photocycloaddition reaction, which has been explored for its ability to produce chiral derivatives with high yields and selectivity . The compound exhibits typical aldehyde reactivity, enabling it to participate in nucleophilic addition reactions, which are crucial for its role as a building block in synthetic chemistry.
Biological Activity
Recent studies have indicated that CBCA may possess significant biological activities, particularly in the context of drug development. Its potential applications include:
- Neurological Disorders : Research suggests that CBCA could serve as a precursor for novel drug candidates aimed at treating conditions such as pain management and neurological disorders.
- Anti-inflammatory Properties : Preliminary findings indicate that CBCA may exhibit anti-inflammatory effects, although further research is needed to fully understand its pharmacological profile.
- Histone Deacetylase Inhibition : Some studies have explored the role of compounds similar to CBCA in inhibiting histone deacetylases (HDACs), which are important targets in cancer therapy. The structure-activity relationship (SAR) studies indicate that modifications to the carbonyl group can enhance HDAC inhibition, suggesting that CBCA derivatives may also possess similar properties .
1. Structure-Activity Relationship Studies
A study focused on hydrazide-based compounds demonstrated that structural modifications could lead to potent HDAC inhibitors. Although not directly related to CBCA, the findings emphasize the importance of structural dynamics in developing effective therapeutics .
2. Conformational Dynamics
Research on the conformational dynamics of CBCA has revealed insights into its vibrational modes and potential energy surfaces. Understanding these dynamics is crucial for predicting the compound's behavior in biological systems and its interactions with various targets .
Comparative Analysis
To highlight the uniqueness of CBCA among similar compounds, the following table compares it with related aldehydes:
Compound Name | Molecular Formula | Unique Features |
---|---|---|
This compound (CBCA) | C4H6O | Four-membered ring; potential anti-inflammatory activity |
Cyclopentanecarboxaldehyde | C5H8O | Five-membered ring; more flexible |
Cyclohexanecarboxaldehyde | C6H10O | Larger ring structure; different reactivity |
1-Cyclopropanecarboxaldehyde | C4H6O | Three-membered ring; higher strain energy |
Properties
IUPAC Name |
cyclobutanecarbaldehyde | |
---|---|---|
Source | PubChem | |
URL | https://pubchem.ncbi.nlm.nih.gov | |
Description | Data deposited in or computed by PubChem | |
InChI |
InChI=1S/C5H8O/c6-4-5-2-1-3-5/h4-5H,1-3H2 | |
Source | PubChem | |
URL | https://pubchem.ncbi.nlm.nih.gov | |
Description | Data deposited in or computed by PubChem | |
InChI Key |
INVYSLWXPIEDIQ-UHFFFAOYSA-N | |
Source | PubChem | |
URL | https://pubchem.ncbi.nlm.nih.gov | |
Description | Data deposited in or computed by PubChem | |
Canonical SMILES |
C1CC(C1)C=O | |
Source | PubChem | |
URL | https://pubchem.ncbi.nlm.nih.gov | |
Description | Data deposited in or computed by PubChem | |
Molecular Formula |
C5H8O | |
Source | PubChem | |
URL | https://pubchem.ncbi.nlm.nih.gov | |
Description | Data deposited in or computed by PubChem | |
DSSTOX Substance ID |
DTXSID70498011 | |
Record name | Cyclobutanecarbaldehyde | |
Source | EPA DSSTox | |
URL | https://comptox.epa.gov/dashboard/DTXSID70498011 | |
Description | DSSTox provides a high quality public chemistry resource for supporting improved predictive toxicology. | |
Molecular Weight |
84.12 g/mol | |
Source | PubChem | |
URL | https://pubchem.ncbi.nlm.nih.gov | |
Description | Data deposited in or computed by PubChem | |
CAS No. |
2987-17-9 | |
Record name | Cyclobutanecarboxaldehyde | |
Source | CAS Common Chemistry | |
URL | https://commonchemistry.cas.org/detail?cas_rn=2987-17-9 | |
Description | CAS Common Chemistry is an open community resource for accessing chemical information. Nearly 500,000 chemical substances from CAS REGISTRY cover areas of community interest, including common and frequently regulated chemicals, and those relevant to high school and undergraduate chemistry classes. This chemical information, curated by our expert scientists, is provided in alignment with our mission as a division of the American Chemical Society. | |
Explanation | The data from CAS Common Chemistry is provided under a CC-BY-NC 4.0 license, unless otherwise stated. | |
Record name | Cyclobutanecarbaldehyde | |
Source | EPA DSSTox | |
URL | https://comptox.epa.gov/dashboard/DTXSID70498011 | |
Description | DSSTox provides a high quality public chemistry resource for supporting improved predictive toxicology. | |
Record name | Cyclobutanecarboxaldehyde | |
Source | European Chemicals Agency (ECHA) | |
URL | https://echa.europa.eu/substance-information/-/substanceinfo/100.119.512 | |
Description | The European Chemicals Agency (ECHA) is an agency of the European Union which is the driving force among regulatory authorities in implementing the EU's groundbreaking chemicals legislation for the benefit of human health and the environment as well as for innovation and competitiveness. | |
Explanation | Use of the information, documents and data from the ECHA website is subject to the terms and conditions of this Legal Notice, and subject to other binding limitations provided for under applicable law, the information, documents and data made available on the ECHA website may be reproduced, distributed and/or used, totally or in part, for non-commercial purposes provided that ECHA is acknowledged as the source: "Source: European Chemicals Agency, http://echa.europa.eu/". Such acknowledgement must be included in each copy of the material. ECHA permits and encourages organisations and individuals to create links to the ECHA website under the following cumulative conditions: Links can only be made to webpages that provide a link to the Legal Notice page. | |
Retrosynthesis Analysis
AI-Powered Synthesis Planning: Our tool employs the Template_relevance Pistachio, Template_relevance Bkms_metabolic, Template_relevance Pistachio_ringbreaker, Template_relevance Reaxys, Template_relevance Reaxys_biocatalysis model, leveraging a vast database of chemical reactions to predict feasible synthetic routes.
One-Step Synthesis Focus: Specifically designed for one-step synthesis, it provides concise and direct routes for your target compounds, streamlining the synthesis process.
Accurate Predictions: Utilizing the extensive PISTACHIO, BKMS_METABOLIC, PISTACHIO_RINGBREAKER, REAXYS, REAXYS_BIOCATALYSIS database, our tool offers high-accuracy predictions, reflecting the latest in chemical research and data.
Strategy Settings
Precursor scoring | Relevance Heuristic |
---|---|
Min. plausibility | 0.01 |
Model | Template_relevance |
Template Set | Pistachio/Bkms_metabolic/Pistachio_ringbreaker/Reaxys/Reaxys_biocatalysis |
Top-N result to add to graph | 6 |
Feasible Synthetic Routes
Disclaimer and Information on In-Vitro Research Products
Please be aware that all articles and product information presented on BenchChem are intended solely for informational purposes. The products available for purchase on BenchChem are specifically designed for in-vitro studies, which are conducted outside of living organisms. In-vitro studies, derived from the Latin term "in glass," involve experiments performed in controlled laboratory settings using cells or tissues. It is important to note that these products are not categorized as medicines or drugs, and they have not received approval from the FDA for the prevention, treatment, or cure of any medical condition, ailment, or disease. We must emphasize that any form of bodily introduction of these products into humans or animals is strictly prohibited by law. It is essential to adhere to these guidelines to ensure compliance with legal and ethical standards in research and experimentation.