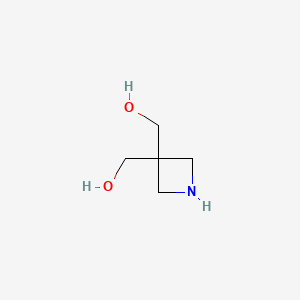
3,3-Di(hydroxymethyl)azetidine
- Click on QUICK INQUIRY to receive a quote from our team of experts.
- With the quality product at a COMPETITIVE price, you can focus more on your research.
Overview
Description
3,3-Di(hydroxymethyl)azetidine, also known as 3,3-bis(hydroxymethyl)azetidine, is a four-membered nitrogen-containing heterocycle. This compound is characterized by the presence of two hydroxymethyl groups attached to the azetidine ring. Azetidines are known for their significant ring strain, which imparts unique reactivity and stability compared to other nitrogen-containing heterocycles .
Mechanism of Action
Target of Action
The primary target of 3,3-Di(hydroxymethyl)azetidine is the low fidelity DNA polymerase Theta (Pol) . This enzyme plays a crucial role in DNA repair and replication, particularly in BRCA-deficient tumors .
Mode of Action
This compound interacts with its target, DNA polymerase Theta, by inhibiting its function . This inhibition is a synthetic-lethal antitumor strategy in BRCA-deficient tumors .
Biochemical Pathways
The inhibition of DNA polymerase Theta affects the DNA repair pathway, particularly in cells compromised by BRCA deficiency . This leads to significant antiproliferative properties in these cells .
Pharmacokinetics
The pharmacokinetics of this compound are favorable, as indicated by the significant antiproliferative properties it exhibits in DNA repair-compromised cells . The compound is metabolically stable, which contributes to its bioavailability .
Result of Action
The result of this compound’s action is significant antiproliferative properties in DNA repair-compromised cells . This is due to the inhibition of DNA polymerase Theta, which is crucial for DNA repair and replication .
Action Environment
The stability of the compound suggests that it may be resistant to various environmental conditions .
Biochemical Analysis
Biochemical Properties
3,3-Di(hydroxymethyl)azetidine plays a significant role in various biochemical reactions. Its reactivity is driven by the considerable ring strain of the azetidine ring, which makes it more stable than related aziridines . This compound interacts with several enzymes and proteins, including those involved in drug discovery and polymerization processes . The hydroxymethyl groups provide sites for further functionalization, allowing for the formation of various derivatives that can interact with biomolecules through hydrogen bonding and other non-covalent interactions.
Cellular Effects
This compound influences various cellular processes, including cell signaling pathways, gene expression, and cellular metabolism. Its interaction with cellular proteins can lead to changes in cell function, such as alterations in enzyme activity and modulation of metabolic pathways . The compound’s ability to form hydrogen bonds with biomolecules allows it to affect cellular processes at multiple levels, potentially leading to changes in cell proliferation and differentiation.
Molecular Mechanism
At the molecular level, this compound exerts its effects through binding interactions with biomolecules. The hydroxymethyl groups can form hydrogen bonds with amino acid residues in proteins, leading to enzyme inhibition or activation . Additionally, the azetidine ring’s strain-driven reactivity allows it to participate in various chemical reactions, further influencing gene expression and cellular function.
Temporal Effects in Laboratory Settings
In laboratory settings, the effects of this compound can change over time. The compound’s stability and degradation are influenced by factors such as temperature, pH, and the presence of other chemicals . Long-term studies have shown that this compound can have lasting effects on cellular function, including sustained changes in enzyme activity and gene expression.
Dosage Effects in Animal Models
The effects of this compound vary with different dosages in animal models. At low doses, the compound may have beneficial effects, such as enhancing enzyme activity and promoting cell proliferation . At high doses, it can exhibit toxic or adverse effects, including cell death and tissue damage. Threshold effects have been observed, indicating that there is a critical concentration above which the compound’s toxicity significantly increases.
Metabolic Pathways
This compound is involved in several metabolic pathways, interacting with enzymes and cofactors that facilitate its conversion into various metabolites . The compound’s hydroxymethyl groups can undergo oxidation and reduction reactions, affecting metabolic flux and metabolite levels. These interactions can influence the overall metabolic state of the cell, leading to changes in energy production and biosynthesis.
Transport and Distribution
Within cells and tissues, this compound is transported and distributed through interactions with transporters and binding proteins . These interactions help localize the compound to specific cellular compartments, where it can exert its biochemical effects. The compound’s distribution is influenced by its hydrophilicity, allowing it to accumulate in aqueous environments within the cell.
Subcellular Localization
This compound is localized to specific subcellular compartments, including the cytoplasm and organelles such as the endoplasmic reticulum and mitochondria . Its subcellular localization is directed by targeting signals and post-translational modifications that guide the compound to its site of action. This localization is crucial for its activity, as it allows the compound to interact with specific biomolecules and participate in localized biochemical reactions.
Preparation Methods
Synthetic Routes and Reaction Conditions
The synthesis of 3,3-Di(hydroxymethyl)azetidine typically involves the cyclization of suitable precursors under controlled conditions. One common method includes the reaction of azetidine with formaldehyde in the presence of a base, such as potassium carbonate, at elevated temperatures. The reaction is carried out in a solvent system like acetonitrile and methanol in a 9:1 ratio .
Industrial Production Methods
Industrial production of this compound may involve large-scale batch or continuous processes. These methods often utilize optimized reaction conditions to maximize yield and purity. The use of catalysts and advanced purification techniques, such as crystallization and chromatography, are common in industrial settings to ensure the quality of the final product .
Chemical Reactions Analysis
Types of Reactions
3,3-Di(hydroxymethyl)azetidine undergoes various chemical reactions, including:
Oxidation: The hydroxymethyl groups can be oxidized to form aldehydes or carboxylic acids.
Reduction: The compound can be reduced to form the corresponding azetidine derivatives.
Substitution: The hydroxymethyl groups can be substituted with other functional groups through nucleophilic substitution reactions.
Common Reagents and Conditions
Oxidation: Common oxidizing agents include potassium permanganate and chromium trioxide.
Reduction: Reducing agents such as lithium aluminum hydride or sodium borohydride are used.
Substitution: Nucleophiles like alkyl halides or amines are employed under basic conditions.
Major Products Formed
Oxidation: Formation of 3,3-diformylazetidine or 3,3-dicarboxyazetidine.
Reduction: Formation of 3,3-dimethylazetidine.
Substitution: Formation of various substituted azetidines depending on the nucleophile used.
Scientific Research Applications
3,3-Di(hydroxymethyl)azetidine has several applications in scientific research:
Chemistry: Used as a building block in organic synthesis and polymer chemistry.
Biology: Investigated for its potential as a scaffold in drug design and development.
Medicine: Explored for its bioactive properties and potential therapeutic applications.
Industry: Utilized in the synthesis of specialty chemicals and materials
Comparison with Similar Compounds
Similar Compounds
3-Hydroxymethyl-azetidine: A related compound with a single hydroxymethyl group.
Azetidine-2-carboxylic acid: A naturally occurring azetidine derivative found in sugar beets.
2-Azetidinone: A structurally related compound known for its use in β-lactam antibiotics.
Uniqueness
3,3-Di(hydroxymethyl)azetidine is unique due to the presence of two hydroxymethyl groups, which enhance its reactivity and potential for functionalization. This makes it a valuable intermediate in organic synthesis and a promising candidate for various applications in medicinal chemistry and materials science .
Properties
IUPAC Name |
[3-(hydroxymethyl)azetidin-3-yl]methanol |
Source
|
---|---|---|
Source | PubChem | |
URL | https://pubchem.ncbi.nlm.nih.gov | |
Description | Data deposited in or computed by PubChem | |
InChI |
InChI=1S/C5H11NO2/c7-3-5(4-8)1-6-2-5/h6-8H,1-4H2 |
Source
|
Source | PubChem | |
URL | https://pubchem.ncbi.nlm.nih.gov | |
Description | Data deposited in or computed by PubChem | |
InChI Key |
JAXQPBINKGFTPK-UHFFFAOYSA-N |
Source
|
Source | PubChem | |
URL | https://pubchem.ncbi.nlm.nih.gov | |
Description | Data deposited in or computed by PubChem | |
Canonical SMILES |
C1C(CN1)(CO)CO |
Source
|
Source | PubChem | |
URL | https://pubchem.ncbi.nlm.nih.gov | |
Description | Data deposited in or computed by PubChem | |
Molecular Formula |
C5H11NO2 |
Source
|
Source | PubChem | |
URL | https://pubchem.ncbi.nlm.nih.gov | |
Description | Data deposited in or computed by PubChem | |
Molecular Weight |
117.15 g/mol |
Source
|
Source | PubChem | |
URL | https://pubchem.ncbi.nlm.nih.gov | |
Description | Data deposited in or computed by PubChem | |
Disclaimer and Information on In-Vitro Research Products
Please be aware that all articles and product information presented on BenchChem are intended solely for informational purposes. The products available for purchase on BenchChem are specifically designed for in-vitro studies, which are conducted outside of living organisms. In-vitro studies, derived from the Latin term "in glass," involve experiments performed in controlled laboratory settings using cells or tissues. It is important to note that these products are not categorized as medicines or drugs, and they have not received approval from the FDA for the prevention, treatment, or cure of any medical condition, ailment, or disease. We must emphasize that any form of bodily introduction of these products into humans or animals is strictly prohibited by law. It is essential to adhere to these guidelines to ensure compliance with legal and ethical standards in research and experimentation.