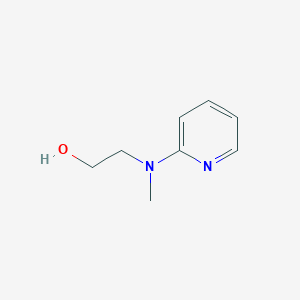
2-(Methyl(pyridin-2-yl)amino)ethanol
Overview
Description
2-(Methyl(pyridin-2-yl)amino)ethanol is an organic compound with the molecular formula C8H12N2O. It is a derivative of pyridine, a heterocyclic aromatic organic compound. This compound is known for its applications in various fields, including medicinal chemistry and organic synthesis .
Mechanism of Action
Mode of Action
It is known that pyridine derivatives can interact with various biological targets through non-covalent interactions such as hydrogen bonding and π-π stacking .
Biochemical Pathways
Pyridine derivatives are known to participate in a variety of biochemical reactions, but the exact pathways influenced by this particular compound require further investigation .
Biochemical Analysis
Biochemical Properties
It is known that pyridine derivatives can react with aryl and alkenyl chlorides in palladium-catalyzed reactions, resulting in the substitution of the chloro moieties with a 2-pyridylmethyl group .
Cellular Effects
It is known that pyridine derivatives can have various effects on cells, including influencing cell signaling pathways, gene expression, and cellular metabolism .
Temporal Effects in Laboratory Settings
It is known that the compound is stable under room temperature conditions .
Preparation Methods
Synthetic Routes and Reaction Conditions
The synthesis of 2-(Methyl(pyridin-2-yl)amino)ethanol typically involves the reaction of 2-chloropyridine with methylamine, followed by the addition of ethylene oxide. The reaction conditions often include the use of a solvent such as ethanol and a catalyst like sodium hydroxide to facilitate the reaction .
Industrial Production Methods
In industrial settings, the production of this compound may involve continuous flow processes to ensure high yield and purity. The use of automated reactors and precise control of reaction parameters such as temperature and pressure are crucial for large-scale production .
Chemical Reactions Analysis
Types of Reactions
2-(Methyl(pyridin-2-yl)amino)ethanol undergoes various types of chemical reactions, including:
Oxidation: This compound can be oxidized to form corresponding ketones or aldehydes.
Reduction: Reduction reactions can convert it into amines or alcohols.
Substitution: It can undergo nucleophilic substitution reactions, where the hydroxyl group is replaced by other functional groups.
Common Reagents and Conditions
Oxidation: Common oxidizing agents include potassium permanganate (KMnO4) and chromium trioxide (CrO3).
Reduction: Reducing agents such as lithium aluminum hydride (LiAlH4) and sodium borohydride (NaBH4) are often used.
Substitution: Reagents like thionyl chloride (SOCl2) and phosphorus tribromide (PBr3) are employed for substitution reactions.
Major Products Formed
Oxidation: Formation of pyridine carboxylic acids.
Reduction: Production of pyridine-based alcohols and amines.
Substitution: Generation of various substituted pyridine derivatives.
Scientific Research Applications
2-(Methyl(pyridin-2-yl)amino)ethanol has a wide range of applications in scientific research:
Chemistry: It is used as a building block in the synthesis of complex organic molecules.
Biology: The compound is employed in the study of enzyme mechanisms and protein-ligand interactions.
Medicine: It serves as a precursor in the synthesis of pharmaceutical agents, including drugs for treating metabolic disorders.
Industry: It is utilized in the production of agrochemicals and specialty chemicals
Comparison with Similar Compounds
Similar Compounds
2-(Pyridin-2-yl)ethanol: Similar in structure but lacks the methyl group on the nitrogen atom.
2-(Pyridin-2-yl)ethylamine: Contains an amine group instead of a hydroxyl group.
2-(Methyl(pyridin-2-yl)amino)propane: Similar structure with a propane chain instead of an ethanol chain.
Uniqueness
2-(Methyl(pyridin-2-yl)amino)ethanol is unique due to its specific combination of functional groups, which allows it to participate in a variety of chemical reactions and interact with diverse molecular targets. This versatility makes it valuable in multiple research and industrial applications .
Properties
IUPAC Name |
2-[methyl(pyridin-2-yl)amino]ethanol | |
---|---|---|
Source | PubChem | |
URL | https://pubchem.ncbi.nlm.nih.gov | |
Description | Data deposited in or computed by PubChem | |
InChI |
InChI=1S/C8H12N2O/c1-10(6-7-11)8-4-2-3-5-9-8/h2-5,11H,6-7H2,1H3 | |
Source | PubChem | |
URL | https://pubchem.ncbi.nlm.nih.gov | |
Description | Data deposited in or computed by PubChem | |
InChI Key |
MWGKOPUDDQZERY-UHFFFAOYSA-N | |
Source | PubChem | |
URL | https://pubchem.ncbi.nlm.nih.gov | |
Description | Data deposited in or computed by PubChem | |
Canonical SMILES |
CN(CCO)C1=CC=CC=N1 | |
Source | PubChem | |
URL | https://pubchem.ncbi.nlm.nih.gov | |
Description | Data deposited in or computed by PubChem | |
Molecular Formula |
C8H12N2O | |
Source | PubChem | |
URL | https://pubchem.ncbi.nlm.nih.gov | |
Description | Data deposited in or computed by PubChem | |
DSSTOX Substance ID |
DTXSID70353237 | |
Record name | 2-[Methyl(pyridin-2-yl)amino]ethan-1-ol | |
Source | EPA DSSTox | |
URL | https://comptox.epa.gov/dashboard/DTXSID70353237 | |
Description | DSSTox provides a high quality public chemistry resource for supporting improved predictive toxicology. | |
Molecular Weight |
152.19 g/mol | |
Source | PubChem | |
URL | https://pubchem.ncbi.nlm.nih.gov | |
Description | Data deposited in or computed by PubChem | |
CAS No. |
122321-04-4 | |
Record name | 2-[N-Methyl-N-(2-pyridyl)amino]ethanol | |
Source | CAS Common Chemistry | |
URL | https://commonchemistry.cas.org/detail?cas_rn=122321-04-4 | |
Description | CAS Common Chemistry is an open community resource for accessing chemical information. Nearly 500,000 chemical substances from CAS REGISTRY cover areas of community interest, including common and frequently regulated chemicals, and those relevant to high school and undergraduate chemistry classes. This chemical information, curated by our expert scientists, is provided in alignment with our mission as a division of the American Chemical Society. | |
Explanation | The data from CAS Common Chemistry is provided under a CC-BY-NC 4.0 license, unless otherwise stated. | |
Record name | 2-[Methyl(pyridin-2-yl)amino]ethan-1-ol | |
Source | EPA DSSTox | |
URL | https://comptox.epa.gov/dashboard/DTXSID70353237 | |
Description | DSSTox provides a high quality public chemistry resource for supporting improved predictive toxicology. | |
Record name | Ethanol, 2-(methyl-2-pyridinylamino) | |
Source | European Chemicals Agency (ECHA) | |
URL | https://echa.europa.eu/substance-information/-/substanceinfo/100.121.493 | |
Description | The European Chemicals Agency (ECHA) is an agency of the European Union which is the driving force among regulatory authorities in implementing the EU's groundbreaking chemicals legislation for the benefit of human health and the environment as well as for innovation and competitiveness. | |
Explanation | Use of the information, documents and data from the ECHA website is subject to the terms and conditions of this Legal Notice, and subject to other binding limitations provided for under applicable law, the information, documents and data made available on the ECHA website may be reproduced, distributed and/or used, totally or in part, for non-commercial purposes provided that ECHA is acknowledged as the source: "Source: European Chemicals Agency, http://echa.europa.eu/". Such acknowledgement must be included in each copy of the material. ECHA permits and encourages organisations and individuals to create links to the ECHA website under the following cumulative conditions: Links can only be made to webpages that provide a link to the Legal Notice page. | |
Synthesis routes and methods I
Procedure details
Synthesis routes and methods II
Procedure details
Retrosynthesis Analysis
AI-Powered Synthesis Planning: Our tool employs the Template_relevance Pistachio, Template_relevance Bkms_metabolic, Template_relevance Pistachio_ringbreaker, Template_relevance Reaxys, Template_relevance Reaxys_biocatalysis model, leveraging a vast database of chemical reactions to predict feasible synthetic routes.
One-Step Synthesis Focus: Specifically designed for one-step synthesis, it provides concise and direct routes for your target compounds, streamlining the synthesis process.
Accurate Predictions: Utilizing the extensive PISTACHIO, BKMS_METABOLIC, PISTACHIO_RINGBREAKER, REAXYS, REAXYS_BIOCATALYSIS database, our tool offers high-accuracy predictions, reflecting the latest in chemical research and data.
Strategy Settings
Precursor scoring | Relevance Heuristic |
---|---|
Min. plausibility | 0.01 |
Model | Template_relevance |
Template Set | Pistachio/Bkms_metabolic/Pistachio_ringbreaker/Reaxys/Reaxys_biocatalysis |
Top-N result to add to graph | 6 |
Feasible Synthetic Routes
Q1: What are the improved synthesis methods for 2-(Methyl(pyridin-2-yl)amino)ethanol and why are they advantageous?
A1: Two improved synthesis methods have been reported []:
Q2: How is the structure of the synthesized 4-[2-(methyl-pyridin-2-yl-amino)-ethoxy]-benzaldehyde confirmed?
A2: The structure of the synthesized 4-[2-(methyl-pyridin-2-yl-amino)-ethoxy]-benzaldehyde is confirmed using spectroscopic techniques: proton Nuclear Magnetic Resonance (¹HNMR) and Mass Spectrometry (MS) []. These methods provide detailed information about the compound's structure and molecular weight, confirming its successful synthesis.
Disclaimer and Information on In-Vitro Research Products
Please be aware that all articles and product information presented on BenchChem are intended solely for informational purposes. The products available for purchase on BenchChem are specifically designed for in-vitro studies, which are conducted outside of living organisms. In-vitro studies, derived from the Latin term "in glass," involve experiments performed in controlled laboratory settings using cells or tissues. It is important to note that these products are not categorized as medicines or drugs, and they have not received approval from the FDA for the prevention, treatment, or cure of any medical condition, ailment, or disease. We must emphasize that any form of bodily introduction of these products into humans or animals is strictly prohibited by law. It is essential to adhere to these guidelines to ensure compliance with legal and ethical standards in research and experimentation.