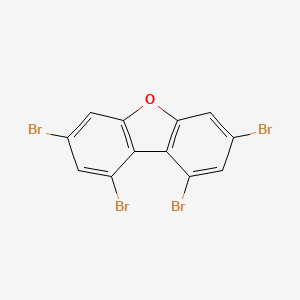
1,3,7,9-Tetrabromo-dibenzofuran
- Click on QUICK INQUIRY to receive a quote from our team of experts.
- With the quality product at a COMPETITIVE price, you can focus more on your research.
Overview
Description
1,3,7,9-Tetrabromo-dibenzofuran (C₁₂H₄Br₄O, molecular weight: 483.776 g/mol) is a brominated aromatic heterocyclic compound characterized by a dibenzofuran backbone substituted with four bromine atoms at the 1, 3, 7, and 9 positions . Its IUPAC InChIKey (PFKOPXBJSVTQLW-UHFFFAOYSA-N) reflects its distinct substitution pattern, which influences its physicochemical properties, environmental persistence, and reactivity.
Preparation Methods
Synthetic Routes and Reaction Conditions
The synthesis of 1,3,7,9-Tetrabromo-dibenzofuran typically involves the bromination of dibenzofuran. One common method is the direct bromination of dibenzofuran using bromine in the presence of a catalyst such as iron or aluminum bromide. The reaction is carried out under controlled conditions to ensure selective bromination at the 1,3,7,9 positions .
Industrial Production Methods
Industrial production of this compound follows similar synthetic routes but on a larger scale. The process involves the use of large reactors and precise control of reaction parameters to achieve high yields and purity. The bromination reaction is typically conducted in a solvent such as chloroform or carbon tetrachloride to facilitate the reaction and improve product isolation .
Chemical Reactions Analysis
Types of Reactions
1,3,7,9-Tetrabromo-dibenzofuran undergoes various chemical reactions, including:
Substitution Reactions: The bromine atoms can be substituted by other nucleophiles such as hydroxyl, amino, or alkyl groups.
Reduction Reactions: The bromine atoms can be reduced to hydrogen using reducing agents like zinc and hydrochloric acid.
Oxidation Reactions: The compound can be oxidized to form dibenzofuran derivatives with different functional groups.
Common Reagents and Conditions
Substitution: Nucleophiles such as sodium hydroxide or ammonia in aqueous or alcoholic solutions.
Reduction: Zinc dust and hydrochloric acid under reflux conditions.
Oxidation: Potassium permanganate or chromium trioxide in acidic or basic media.
Major Products Formed
Substitution: Hydroxylated or aminated dibenzofuran derivatives.
Reduction: Dibenzofuran.
Oxidation: Dibenzofuran derivatives with carboxyl or aldehyde groups.
Scientific Research Applications
1,3,7,9-Tetrabromo-dibenzofuran has several applications in scientific research:
Chemistry: Used as a precursor for the synthesis of other brominated organic compounds and as a reagent in organic synthesis.
Biology: Studied for its potential biological activities, including antimicrobial and anticancer properties.
Medicine: Investigated for its potential use in drug development, particularly for its ability to interact with biological targets.
Industry: Used in the production of flame retardants and other brominated materials
Mechanism of Action
The mechanism of action of 1,3,7,9-Tetrabromo-dibenzofuran involves its interaction with molecular targets such as enzymes and receptors. The bromine atoms enhance the compound’s ability to form strong interactions with these targets, leading to various biological effects. The pathways involved may include inhibition of enzyme activity or modulation of receptor signaling .
Comparison with Similar Compounds
Structural Isomers: 2,3,7,8-Tetrabromo-dibenzofuran
The isomer 2,3,7,8-tetrabromo-dibenzofuran (C₁₂H₄Br₄O, molecular weight: 483.776 g/mol) shares the same molecular formula but differs in bromine substitution positions (2,3,7,8 vs. 1,3,7,9) . This positional isomerism significantly impacts properties:
- Symmetry and Stability : The 1,3,7,9 isomer exhibits a symmetrical substitution pattern, which may enhance thermodynamic stability compared to the asymmetrical 2,3,7,8 isomer.
- Formation Pathways : Studies on polychlorinated analogs suggest that symmetrical substitution (e.g., 1,3,6,8-TeCTA) requires higher activation energy (13.60 kcal/mol) than asymmetrical pathways (12.69 kcal/mol for 1,3,7,9-TeCTA), implying kinetic favorability for asymmetrical products .
Property | 1,3,7,9-Tetrabromo-dibenzofuran | 2,3,7,8-Tetrabromo-dibenzofuran |
---|---|---|
Molecular Weight (g/mol) | 483.776 | 483.776 |
Substitution Pattern | 1,3,7,9 | 2,3,7,8 |
Symmetry | Symmetrical | Asymmetrical |
logPoct/wat | Not reported | Not reported |
Chlorinated Analogs: 2,3,7,8-Tetrachloro-dibenzofuran
Chlorinated analogs like 2,3,7,8-tetrachloro-dibenzofuran (C₁₂H₄Cl₄O, CAS: 51207-31-9) exhibit similar environmental persistence but differ in key aspects :
- Polarizability and Bioaccumulation : Bromine’s larger atomic radius increases van der Waals interactions, leading to higher logP values (e.g., 6.636 for 1,2,8,9-tetrabromo-dibenzofuran ) compared to chlorinated analogs.
- Toxicity : Chlorinated dioxins (e.g., TCDD) are more extensively studied for toxicity, but brominated analogs may exhibit comparable or unique endocrine-disrupting effects due to heavier halogenation.
Higher Brominated Congeners: 1,2,3,7,9-Pentabromo-dibenzofuran
However, steric hindrance from additional bromine atoms may reduce reactivity in certain environmental degradation processes.
Functionalized Derivatives: Hydroxylated and Methylated Dibenzofurans
A derivative isolated from Antarctic lichen, 1,3,7,9-tetrahydroxy-2,8-dimethyl-4,6-di(ethanoyl)dibenzofuran, highlights how substituent groups alter properties :
- Solubility : Hydroxyl groups increase aqueous solubility, contrasting with the lipophilicity of brominated analogs.
- Bioactivity: Structural modifications (e.g., enamino diketo groups in synthetic dibenzofurans) can confer immunosuppressive activity, suggesting brominated derivatives may have unexplored biological roles .
Key Research Findings
- Formation Mechanisms : this compound forms via Smiles rearrangement pathways with a lower activation barrier (12.69 kcal/mol) than its symmetrical isomers, making it kinetically favorable .
- Analytical Challenges : Isotope-labeled analogs (e.g., <sup>13</sup>C-tetrabromo-diphenyl ether ) are critical for tracking environmental transport and degradation of brominated dibenzofurans.
Biological Activity
1,3,7,9-Tetrabromo-dibenzofuran (TBD) is a halogenated derivative of dibenzofuran characterized by the presence of four bromine atoms. Its chemical formula is C₁₂H₄Br₄O, and it has a molecular weight of approximately 483.776 g/mol. This compound has garnered attention due to its significant biological activity and potential environmental implications, particularly as a persistent organic pollutant.
The biological activity of TBD is primarily attributed to its interaction with the aryl hydrocarbon receptor (AhR). This receptor is involved in the regulation of xenobiotic metabolism and can influence various biological processes, including endocrine disruption. The presence of bromine atoms enhances TBD's ability to form strong interactions with molecular targets such as enzymes and receptors, leading to various biological effects including:
- Enzyme Inhibition : TBD may inhibit specific enzyme activities, affecting metabolic pathways.
- Receptor Modulation : It can modulate signaling pathways associated with hormone receptors.
Biological Activities
Research indicates that TBD exhibits several notable biological activities:
- Antimicrobial Properties : TBD has been studied for its potential to inhibit the growth of various microbial strains.
- Anticancer Activity : Preliminary studies suggest that TBD may possess anticancer properties, although detailed mechanisms remain under investigation.
Comparative Analysis with Similar Compounds
TBD shares structural similarities with other brominated dibenzofurans. The following table summarizes key characteristics and unique features of TBD compared to related compounds:
Compound Name | Formula | Unique Features |
---|---|---|
2,3,7,8-Tetrabromodibenzofuran | C₁₂H₄Br₄O | Higher toxicity and environmental persistence |
2,3-Dibromodibenzofuran | C₁₂H₈Br₂O | Fewer bromine substituents lead to different reactivity |
2,3,7-Triiododibenzofuran | C₁₂H₄I₃O | Contains iodine instead of bromine; different interactions |
Case Studies and Research Findings
Several studies have explored the biological activity and environmental impact of TBD:
- Bioaccumulation Studies : A study conducted on C57BL/6J mice examined the uptake and elimination kinetics of brominated dibenzofurans. The results indicated that dibenzofurans exhibit varying degrees of bioaccumulation potential, with TBD showing lower hepatic uptake ratios compared to more toxic compounds like TCDD (2,3,7,8-tetrachlorodibenzo-p-dioxin) .
- Toxicological Assessments : In a comparative assessment involving various brominated compounds, TBD was found to activate AhR-dependent pathways similarly to other halogenated compounds. This suggests potential toxicological effects that warrant further investigation into its safety profile .
- Environmental Impact Studies : Research has highlighted the persistence of TBD in environmental samples, raising concerns about its accumulation in food chains through feed additives . The compound was detected in poultry feed and eggs, indicating possible routes of human exposure .
Q & A
Basic Research Questions
Q. What are the primary methodologies for synthesizing 1,3,7,9-tetrabromo-dibenzofuran derivatives, and how do reaction conditions influence yields?
Methodological Answer: Synthesis of brominated dibenzofurans often involves electrophilic substitution or halogenation of the parent dibenzofuran structure. For example, ring-opening reactions using sodium metal (Na) under controlled conditions (e.g., 70°C for 6 hours) can yield derivatives like o-phenylphenol with >99.5% purity and 72% yield . Key variables include:
- Temperature : Higher temperatures (e.g., 70°C vs. ambient) accelerate reaction kinetics but may increase side-product formation.
- Substrate concentration : Optimal molar ratios (e.g., 1.08 equivalents of dibenzofuran) minimize unreacted precursors.
- Feeding mode : Batch addition ensures homogeneous mixing, critical for reproducibility.
Table 1: Synthesis Optimization Parameters
Parameter | Optimal Value | Yield/Purity Impact |
---|---|---|
Temperature | 70°C | Maximizes conversion |
Reaction Time | 6 hours | Balances kinetics |
Dibenzofuran:Metal Ratio | 1.08:1 | Minimizes residuals |
Q. How are thermochemical properties (e.g., enthalpy of sublimation) experimentally determined for brominated dibenzofurans?
Methodological Answer: Static bomb calorimetry and vacuum drop microcalorimetry are standard for measuring thermochemical data. For dibenzofuran derivatives:
- Enthalpy of sublimation : Determined as 84.5±1.0kJ mol−1 at 298.15 K using vacuum drop microcalorimetry .
- Standard molar enthalpy of formation : Calculated via combustion calorimetry (e.g., −29.2±3.8kJ mol−1 for crystalline dibenzofuran) .
- Validation : G3(MP2)//B3LYP computational methods align with experimental data within ±3.9 kJ mol−1 .
Table 2: Thermochemical Data for Dibenzofuran Derivatives
Property | Experimental Value | Computational Value |
---|---|---|
Sublimation Enthalpy (kJ/mol) | 84.5±1.0 | 81.2±2.5 |
Gas-phase Formation Enthalpy | 55.0±3.9 | 58.3±4.1 |
Q. What analytical techniques are recommended for quantifying trace levels of this compound in environmental samples?
Methodological Answer: Gas chromatography-mass spectrometry (GC-MS) with NIST library cross-referencing is preferred for identification and quantification. Key steps include:
- Calibration curves : Span a dynamic range with R2>0.98 for linearity validation .
- Sample preparation : Solid-phase extraction (SPE) or liquid-liquid extraction (LLE) to isolate analytes from matrices.
- Validation : Use working standards (e.g., 50 µg/mL toluene solutions) to confirm retention times and fragmentation patterns .
Advanced Research Questions
Q. How do kinetic mechanisms differ between pyrolysis and oxidation of dibenzofuran derivatives at high temperatures?
Methodological Answer: Pyrolysis (500–950°C) and oxidation pathways are studied in perfectly stirred reactors. Key findings:
- Pyrolysis : Dominated by radical chain reactions, yielding phenol and benzofuran via C–O bond cleavage .
- Oxidation : Introduces OH radicals, accelerating decomposition into CO, CO2, and smaller hydrocarbons.
- Modeling : Detailed kinetic mechanisms (e.g., elementary steps from jet-stirred reactor data) predict product distributions within 5–10% error .
Table 3: Reaction Conditions and Major Products
Condition | Temperature Range | Major Products | Conversion Efficiency |
---|---|---|---|
Pyrolysis | 700–950°C | Phenol, Benzofuran | 85–92% |
Oxidation | 500–800°C | CO, CO2, Hydrocarbons | 78–88% |
Q. What metabolic pathways are involved in the microbial degradation of brominated dibenzofurans, and how do substituent positions affect biodegradability?
Methodological Answer: Pseudomonas spp. and Rhodococcus opacus strains metabolize dibenzofurans via:
- Dioxygenase-mediated cleavage : Lateral dioxygenation by enzymes like DbfA initiates degradation, forming 2,2',3-trihydroxybiphenyl .
- Substituent effects : Higher bromination (e.g., PeBDF) reduces metabolic rates (M/P=3.5×10−5) compared to tetrabromo derivatives (M/P=4.5×10−4) due to steric hindrance .
Key Enzymes and Pathways:
Salicylic acid pathway : Gentisic acid and catechol intermediates.
Meta-fission : Cleavage of dihydroxylated aromatic rings.
Q. How do adsorption mechanisms of dibenzofuran derivatives on activated carbon vary with surface chemistry?
Methodological Answer: Langmuir isotherm models describe adsorption on Norit RB1 and Monolith carbons:
- Surface diffusion : Dominates rate control, with diffusion coefficients increasing at higher temperatures (e.g., Ds=1.2×10−10m2/s at 25°C vs. 2.8×10−10m2/s at 40°C) .
- Functional groups : Oxygenated surfaces enhance π-π interactions with aromatic rings, improving adsorption capacity by 15–20% .
Table 4: Adsorption Parameters for Activated Carbons
Carbon Type | qmax (mg/g) | KL (L/mg) | Temperature Dependence |
---|---|---|---|
Norit RB1 | 120.5 | 0.045 | Strong |
Monolith | 98.7 | 0.032 | Moderate |
Q. What computational strategies validate thermochemical and kinetic data for brominated dibenzofurans?
Methodological Answer:
- G3(MP2)//B3LYP : Predicts gas-phase formation enthalpies within ±4 kJ mol−1 of experimental values .
- DFT studies : Analyze adsorption energetics (e.g., binding energies of −50.7 kJ mol−1 for dibenzofuran on activated coke) .
- Mechanistic validation : Compare simulated concentration profiles (e.g., sediment diffusion in 13 mm sand caps) with experimental ranges .
Properties
CAS No. |
617707-80-9 |
---|---|
Molecular Formula |
C12H4Br4O |
Molecular Weight |
483.77 g/mol |
IUPAC Name |
1,3,7,9-tetrabromodibenzofuran |
InChI |
InChI=1S/C12H4Br4O/c13-5-1-7(15)11-9(3-5)17-10-4-6(14)2-8(16)12(10)11/h1-4H |
InChI Key |
PFKOPXBJSVTQLW-UHFFFAOYSA-N |
Canonical SMILES |
C1=C(C=C(C2=C1OC3=C2C(=CC(=C3)Br)Br)Br)Br |
Origin of Product |
United States |
Disclaimer and Information on In-Vitro Research Products
Please be aware that all articles and product information presented on BenchChem are intended solely for informational purposes. The products available for purchase on BenchChem are specifically designed for in-vitro studies, which are conducted outside of living organisms. In-vitro studies, derived from the Latin term "in glass," involve experiments performed in controlled laboratory settings using cells or tissues. It is important to note that these products are not categorized as medicines or drugs, and they have not received approval from the FDA for the prevention, treatment, or cure of any medical condition, ailment, or disease. We must emphasize that any form of bodily introduction of these products into humans or animals is strictly prohibited by law. It is essential to adhere to these guidelines to ensure compliance with legal and ethical standards in research and experimentation.