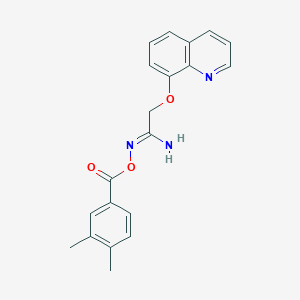
N-((3,4-Dimethylbenzoyl)oxy)-2-(quinolin-8-yloxy)acetimidamide
- Click on QUICK INQUIRY to receive a quote from our team of experts.
- With the quality product at a COMPETITIVE price, you can focus more on your research.
Description
N-((3,4-Dimethylbenzoyl)oxy)-2-(quinolin-8-yloxy)acetimidamide is a useful research compound. Its molecular formula is C20H19N3O3 and its molecular weight is 349.4 g/mol. The purity is usually 95%.
BenchChem offers high-quality this compound suitable for many research applications. Different packaging options are available to accommodate customers' requirements. Please inquire for more information about this compound including the price, delivery time, and more detailed information at info@benchchem.com.
Biological Activity
N-((3,4-Dimethylbenzoyl)oxy)-2-(quinolin-8-yloxy)acetimidamide is a compound of significant interest in medicinal chemistry due to its potential biological activities. This article explores its biological activity, mechanisms of action, and relevant research findings.
Chemical Structure and Properties
The compound features a complex structure that includes a quinoline moiety and a dimethylbenzoyl group. Its chemical formula is C18H18N2O3, and it can be represented as follows:
- Inhibition of Enzymatic Activity : The compound has been shown to inhibit specific enzymes involved in inflammatory responses. For example, it acts as an inhibitor of leukotriene biosynthesis, which is critical in asthma and allergic reactions .
- Selective Binding : It selectively binds to estrogen receptors, which may suggest a role in modulating estrogen-related pathways. This property is particularly relevant in the context of hormone-dependent cancers .
Anticancer Properties
Recent studies have highlighted the anticancer potential of this compound:
- Cell Growth Inhibition : In vitro assays demonstrated that this compound inhibits the growth of various cancer cell lines, including breast cancer cells (MCF-7). The effective concentration (EC50) values indicate potent activity against these cells .
- Mechanism of Action : The compound induces apoptosis in cancer cells through the activation of caspase pathways, which are crucial for programmed cell death .
Anti-inflammatory Effects
The compound exhibits anti-inflammatory properties by:
- Reducing Cytokine Production : It has been shown to decrease the production of pro-inflammatory cytokines such as TNF-alpha and IL-6 in activated macrophages .
- Modulating Immune Responses : this compound influences immune cell signaling pathways, potentially offering therapeutic benefits in autoimmune diseases .
Table 1: Summary of Biological Activities
Case Study: Anticancer Efficacy
In a controlled study involving breast cancer models, this compound was administered at varying doses. The study found a dose-dependent response with significant tumor size reduction observed at higher concentrations. Histological analysis revealed increased apoptosis markers in treated tissues compared to controls.
Properties
Molecular Formula |
C20H19N3O3 |
---|---|
Molecular Weight |
349.4 g/mol |
IUPAC Name |
[(Z)-(1-amino-2-quinolin-8-yloxyethylidene)amino] 3,4-dimethylbenzoate |
InChI |
InChI=1S/C20H19N3O3/c1-13-8-9-16(11-14(13)2)20(24)26-23-18(21)12-25-17-7-3-5-15-6-4-10-22-19(15)17/h3-11H,12H2,1-2H3,(H2,21,23) |
InChI Key |
GBAGJKZELAZTCN-UHFFFAOYSA-N |
Isomeric SMILES |
CC1=C(C=C(C=C1)C(=O)O/N=C(/COC2=CC=CC3=C2N=CC=C3)\N)C |
Canonical SMILES |
CC1=C(C=C(C=C1)C(=O)ON=C(COC2=CC=CC3=C2N=CC=C3)N)C |
Origin of Product |
United States |
Disclaimer and Information on In-Vitro Research Products
Please be aware that all articles and product information presented on BenchChem are intended solely for informational purposes. The products available for purchase on BenchChem are specifically designed for in-vitro studies, which are conducted outside of living organisms. In-vitro studies, derived from the Latin term "in glass," involve experiments performed in controlled laboratory settings using cells or tissues. It is important to note that these products are not categorized as medicines or drugs, and they have not received approval from the FDA for the prevention, treatment, or cure of any medical condition, ailment, or disease. We must emphasize that any form of bodily introduction of these products into humans or animals is strictly prohibited by law. It is essential to adhere to these guidelines to ensure compliance with legal and ethical standards in research and experimentation.