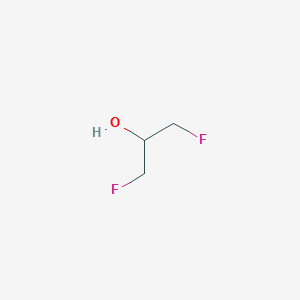
1,3-Difluoro-2-propanol
Overview
Description
1,3-Difluoro-2-propanol is an organic compound with the chemical formula C3H6F2O . It is a metabolic poison that disrupts the citric acid cycle and is used as a rodenticide, similar to sodium fluoroacetate . It is the main ingredient (along with 1-chloro-3-fluoro-2-propanol) in the rodenticide product Gliftor, which was widely used in the former USSR and is still approved in China .
Molecular Structure Analysis
The molecular structure of 1,3-Difluoro-2-propanol consists of three carbon atoms, six hydrogen atoms, two fluorine atoms, and one oxygen atom . The structure is also available as a 2D Mol file or as a computed 3D SD file .Physical And Chemical Properties Analysis
1,3-Difluoro-2-propanol has a molar mass of 96.077 g/mol . It has a density of 1.24 g/cm³ at 25°C . The boiling point of 1,3-Difluoro-2-propanol is between 54 to 55°C .Scientific Research Applications
Synthesis of 1,3-Difluoroacetone
1,3-Difluoro-2-propanol: is utilized in the synthesis of 1,3-difluoroacetone , a compound that serves as an intermediate in organic synthesis . This process is significant for the production of various fluorinated compounds which have applications in pharmaceuticals and agrochemicals.
Rodenticide
As a potent metabolic poison, 1,3-Difluoro-2-propanol disrupts the citric acid cycle. It is a key ingredient in the rodenticide product Gliftor , which has been used extensively in the past in the USSR and is still approved in China . This application is crucial for controlling rodent populations in agricultural and urban settings.
Biochemical Toxicology
The compound’s metabolite, 1,3-difluoroacetone , has been studied for its biochemical toxicology. Specifically, its effects on the respiratory system and potential as a respiratory toxin have been investigated, providing insights into the safety and handling of such compounds .
Research on Antidotes
There has been research into potential antidotes for poisoning by 1,3-Difluoro-2-propanol and its derivatives. Studies have explored compounds like 4-methylpyrazole as potential antidotes, which is important for occupational safety and health in industries that use this chemical .
Mechanism of Action
Target of Action
1,3-Difluoro-2-propanol primarily targets the citric acid cycle , a crucial metabolic pathway in organisms . This cycle is essential for the production of energy in the form of ATP, and disruption of this cycle can lead to significant metabolic disturbances.
Mode of Action
The compound acts as a metabolic poison , disrupting the citric acid cycle . It is similar in action to sodium fluoroacetate, another potent disruptor of the citric acid cycle
Biochemical Pathways
By disrupting the citric acid cycle, 1,3-Difluoro-2-propanol affects a wide range of downstream biochemical pathways. The citric acid cycle is central to cellular respiration, and its disruption can lead to a decrease in ATP production, affecting numerous cellular processes that rely on this vital energy source .
Result of Action
The primary result of 1,3-Difluoro-2-propanol’s action is the disruption of the citric acid cycle, leading to a decrease in ATP production . This can cause a wide range of effects, depending on the extent of exposure and the specific tissues affected. In severe cases, it can lead to metabolic acidosis and death .
Action Environment
The action, efficacy, and stability of 1,3-Difluoro-2-propanol can be influenced by various environmental factors. For example, the compound is used as a rodenticide, and its effectiveness can vary depending on the specific environment in which it is used . .
Safety and Hazards
properties
IUPAC Name |
1,3-difluoropropan-2-ol | |
---|---|---|
Source | PubChem | |
URL | https://pubchem.ncbi.nlm.nih.gov | |
Description | Data deposited in or computed by PubChem | |
InChI |
InChI=1S/C3H6F2O/c4-1-3(6)2-5/h3,6H,1-2H2 | |
Source | PubChem | |
URL | https://pubchem.ncbi.nlm.nih.gov | |
Description | Data deposited in or computed by PubChem | |
InChI Key |
PVDLUGWWIOGCNH-UHFFFAOYSA-N | |
Source | PubChem | |
URL | https://pubchem.ncbi.nlm.nih.gov | |
Description | Data deposited in or computed by PubChem | |
Canonical SMILES |
C(C(CF)O)F | |
Source | PubChem | |
URL | https://pubchem.ncbi.nlm.nih.gov | |
Description | Data deposited in or computed by PubChem | |
Molecular Formula |
C3H6F2O | |
Source | PubChem | |
URL | https://pubchem.ncbi.nlm.nih.gov | |
Description | Data deposited in or computed by PubChem | |
DSSTOX Substance ID |
DTXSID1060009 | |
Record name | 1,3-Difluoro-2-propanol | |
Source | EPA DSSTox | |
URL | https://comptox.epa.gov/dashboard/DTXSID1060009 | |
Description | DSSTox provides a high quality public chemistry resource for supporting improved predictive toxicology. | |
Molecular Weight |
96.08 g/mol | |
Source | PubChem | |
URL | https://pubchem.ncbi.nlm.nih.gov | |
Description | Data deposited in or computed by PubChem | |
Product Name |
1,3-Difluoro-2-propanol | |
CAS RN |
453-13-4 | |
Record name | 1,3-Difluoro-2-propanol | |
Source | CAS Common Chemistry | |
URL | https://commonchemistry.cas.org/detail?cas_rn=453-13-4 | |
Description | CAS Common Chemistry is an open community resource for accessing chemical information. Nearly 500,000 chemical substances from CAS REGISTRY cover areas of community interest, including common and frequently regulated chemicals, and those relevant to high school and undergraduate chemistry classes. This chemical information, curated by our expert scientists, is provided in alignment with our mission as a division of the American Chemical Society. | |
Explanation | The data from CAS Common Chemistry is provided under a CC-BY-NC 4.0 license, unless otherwise stated. | |
Record name | 1,3-Difluoro-2-propanol | |
Source | ChemIDplus | |
URL | https://pubchem.ncbi.nlm.nih.gov/substance/?source=chemidplus&sourceid=0000453134 | |
Description | ChemIDplus is a free, web search system that provides access to the structure and nomenclature authority files used for the identification of chemical substances cited in National Library of Medicine (NLM) databases, including the TOXNET system. | |
Record name | 1,3-Difluoro-2-propanol | |
Source | DTP/NCI | |
URL | https://dtp.cancer.gov/dtpstandard/servlet/dwindex?searchtype=NSC&outputformat=html&searchlist=76034 | |
Description | The NCI Development Therapeutics Program (DTP) provides services and resources to the academic and private-sector research communities worldwide to facilitate the discovery and development of new cancer therapeutic agents. | |
Explanation | Unless otherwise indicated, all text within NCI products is free of copyright and may be reused without our permission. Credit the National Cancer Institute as the source. | |
Record name | 1,3-Difluoro-2-propanol | |
Source | DTP/NCI | |
URL | https://dtp.cancer.gov/dtpstandard/servlet/dwindex?searchtype=NSC&outputformat=html&searchlist=21305 | |
Description | The NCI Development Therapeutics Program (DTP) provides services and resources to the academic and private-sector research communities worldwide to facilitate the discovery and development of new cancer therapeutic agents. | |
Explanation | Unless otherwise indicated, all text within NCI products is free of copyright and may be reused without our permission. Credit the National Cancer Institute as the source. | |
Record name | 2-Propanol, 1,3-difluoro- | |
Source | EPA Chemicals under the TSCA | |
URL | https://www.epa.gov/chemicals-under-tsca | |
Description | EPA Chemicals under the Toxic Substances Control Act (TSCA) collection contains information on chemicals and their regulations under TSCA, including non-confidential content from the TSCA Chemical Substance Inventory and Chemical Data Reporting. | |
Record name | 1,3-Difluoro-2-propanol | |
Source | EPA DSSTox | |
URL | https://comptox.epa.gov/dashboard/DTXSID1060009 | |
Description | DSSTox provides a high quality public chemistry resource for supporting improved predictive toxicology. | |
Record name | 1,3-difluoropropan-2-ol | |
Source | European Chemicals Agency (ECHA) | |
URL | https://echa.europa.eu/substance-information/-/substanceinfo/100.006.561 | |
Description | The European Chemicals Agency (ECHA) is an agency of the European Union which is the driving force among regulatory authorities in implementing the EU's groundbreaking chemicals legislation for the benefit of human health and the environment as well as for innovation and competitiveness. | |
Explanation | Use of the information, documents and data from the ECHA website is subject to the terms and conditions of this Legal Notice, and subject to other binding limitations provided for under applicable law, the information, documents and data made available on the ECHA website may be reproduced, distributed and/or used, totally or in part, for non-commercial purposes provided that ECHA is acknowledged as the source: "Source: European Chemicals Agency, http://echa.europa.eu/". Such acknowledgement must be included in each copy of the material. ECHA permits and encourages organisations and individuals to create links to the ECHA website under the following cumulative conditions: Links can only be made to webpages that provide a link to the Legal Notice page. | |
Record name | 1,3-Difluoro-2-propanol | |
Source | FDA Global Substance Registration System (GSRS) | |
URL | https://gsrs.ncats.nih.gov/ginas/app/beta/substances/R8ZM2LQG7K | |
Description | The FDA Global Substance Registration System (GSRS) enables the efficient and accurate exchange of information on what substances are in regulated products. Instead of relying on names, which vary across regulatory domains, countries, and regions, the GSRS knowledge base makes it possible for substances to be defined by standardized, scientific descriptions. | |
Explanation | Unless otherwise noted, the contents of the FDA website (www.fda.gov), both text and graphics, are not copyrighted. They are in the public domain and may be republished, reprinted and otherwise used freely by anyone without the need to obtain permission from FDA. Credit to the U.S. Food and Drug Administration as the source is appreciated but not required. | |
Retrosynthesis Analysis
AI-Powered Synthesis Planning: Our tool employs the Template_relevance Pistachio, Template_relevance Bkms_metabolic, Template_relevance Pistachio_ringbreaker, Template_relevance Reaxys, Template_relevance Reaxys_biocatalysis model, leveraging a vast database of chemical reactions to predict feasible synthetic routes.
One-Step Synthesis Focus: Specifically designed for one-step synthesis, it provides concise and direct routes for your target compounds, streamlining the synthesis process.
Accurate Predictions: Utilizing the extensive PISTACHIO, BKMS_METABOLIC, PISTACHIO_RINGBREAKER, REAXYS, REAXYS_BIOCATALYSIS database, our tool offers high-accuracy predictions, reflecting the latest in chemical research and data.
Strategy Settings
Precursor scoring | Relevance Heuristic |
---|---|
Min. plausibility | 0.01 |
Model | Template_relevance |
Template Set | Pistachio/Bkms_metabolic/Pistachio_ringbreaker/Reaxys/Reaxys_biocatalysis |
Top-N result to add to graph | 6 |
Feasible Synthetic Routes
Disclaimer and Information on In-Vitro Research Products
Please be aware that all articles and product information presented on BenchChem are intended solely for informational purposes. The products available for purchase on BenchChem are specifically designed for in-vitro studies, which are conducted outside of living organisms. In-vitro studies, derived from the Latin term "in glass," involve experiments performed in controlled laboratory settings using cells or tissues. It is important to note that these products are not categorized as medicines or drugs, and they have not received approval from the FDA for the prevention, treatment, or cure of any medical condition, ailment, or disease. We must emphasize that any form of bodily introduction of these products into humans or animals is strictly prohibited by law. It is essential to adhere to these guidelines to ensure compliance with legal and ethical standards in research and experimentation.