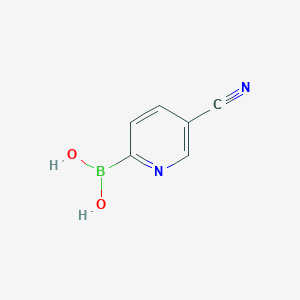
(5-Cyanopyridin-2-yl)boronic acid
- Click on QUICK INQUIRY to receive a quote from our team of experts.
- With the quality product at a COMPETITIVE price, you can focus more on your research.
Overview
Description
Synthesis Analysis
“(5-Cyanopyridin-2-yl)boronic acid” and its esters are synthesized through regioselective halogen-metal exchange methods. These compounds, prepared from dihalopyridines, undergo palladium-catalyzed coupling, forming diverse pyridine libraries.Molecular Structure Analysis
The empirical formula of “(5-Cyanopyridin-2-yl)boronic acid” is C6H5BN2O2 . Its molecular weight is 147.93 . The SMILES string is OB(C1=CC=C(C#N)C=N1)O .Chemical Reactions Analysis
“(5-Cyanopyridin-2-yl)boronic acid” is primarily used as a reagent for the synthesis of various molecules and as a catalyst for various reactions. It has been extensively studied for its potential biochemical and physiological effects, as well as its potential applications in lab experiments.Physical And Chemical Properties Analysis
The empirical formula of “(5-Cyanopyridin-2-yl)boronic acid” is C6H5BN2O2 . Its molecular weight is 147.93 . The SMILES string is OB(C1=CC=C(C#N)C=N1)O .Scientific Research Applications
Organic Synthesis
5-Cyanopyridine-2-boronic acid: is a versatile compound in organic synthesis. It acts as an important intermediate for constructing complex molecules due to its reactive boronic acid group, which can form carbon-carbon bonds through cross-coupling reactions like the Suzuki reaction . This reactivity is pivotal for synthesizing various organic compounds, including pharmaceuticals and agrochemicals.
Pharmaceutical Research
In pharmaceutical research, 5-Cyanopyridine-2-boronic acid serves as a building block for drug development. Its structure is key in the synthesis of molecules that interact with biological targets. For example, it can be used to create ligands for receptors or enzymes, potentially leading to new treatments for diseases .
Agrochemical Production
The compound finds application in the development of agrochemicals. Its boronic acid moiety is instrumental in creating new pesticides and herbicides, offering a way to control agricultural pests and improve crop yields effectively .
Dye Manufacturing
5-Cyanopyridine-2-boronic acid: is also used in the production of dyes. Its chemical structure allows for the introduction of various substituents, enabling the synthesis of dyes with specific properties for textiles and industrial applications .
Sensing Technologies
Boronic acids, including 5-Cyanopyridine-2-boronic acid , are known for their ability to interact with diols and Lewis bases, which makes them useful in sensing applications. They can be employed in the detection of sugars, alcohols, and other substances with diol groups, which is valuable in environmental monitoring and medical diagnostics .
Material Science
In material science, 5-Cyanopyridine-2-boronic acid can be used to modify surfaces or create polymers with specific characteristics. The boronic acid group allows for the formation of stable covalent bonds with other materials, leading to the development of novel materials with enhanced properties .
Biochemical Tool
This compound is a biochemical tool for protein manipulation and modification. It can be used to label proteins or to modify their function, which is crucial in understanding biological processes and developing therapeutic strategies .
Controlled Release Systems
Lastly, 5-Cyanopyridine-2-boronic acid has potential applications in the development of controlled release systems. For instance, it can be incorporated into polymers that respond to the presence of specific biomolecules, leading to the release of active agents like insulin in response to blood sugar levels .
Safety And Hazards
properties
IUPAC Name |
(5-cyanopyridin-2-yl)boronic acid |
Source
|
---|---|---|
Source | PubChem | |
URL | https://pubchem.ncbi.nlm.nih.gov | |
Description | Data deposited in or computed by PubChem | |
InChI |
InChI=1S/C6H5BN2O2/c8-3-5-1-2-6(7(10)11)9-4-5/h1-2,4,10-11H |
Source
|
Source | PubChem | |
URL | https://pubchem.ncbi.nlm.nih.gov | |
Description | Data deposited in or computed by PubChem | |
InChI Key |
BUJDSZUNQGJXOS-UHFFFAOYSA-N |
Source
|
Source | PubChem | |
URL | https://pubchem.ncbi.nlm.nih.gov | |
Description | Data deposited in or computed by PubChem | |
Canonical SMILES |
B(C1=NC=C(C=C1)C#N)(O)O |
Source
|
Source | PubChem | |
URL | https://pubchem.ncbi.nlm.nih.gov | |
Description | Data deposited in or computed by PubChem | |
Molecular Formula |
C6H5BN2O2 |
Source
|
Source | PubChem | |
URL | https://pubchem.ncbi.nlm.nih.gov | |
Description | Data deposited in or computed by PubChem | |
DSSTOX Substance ID |
DTXSID10590543 |
Source
|
Record name | (5-Cyanopyridin-2-yl)boronic acid | |
Source | EPA DSSTox | |
URL | https://comptox.epa.gov/dashboard/DTXSID10590543 | |
Description | DSSTox provides a high quality public chemistry resource for supporting improved predictive toxicology. | |
Molecular Weight |
147.93 g/mol |
Source
|
Source | PubChem | |
URL | https://pubchem.ncbi.nlm.nih.gov | |
Description | Data deposited in or computed by PubChem | |
Product Name |
(5-Cyanopyridin-2-yl)boronic acid | |
CAS RN |
910547-29-4 |
Source
|
Record name | (5-Cyanopyridin-2-yl)boronic acid | |
Source | EPA DSSTox | |
URL | https://comptox.epa.gov/dashboard/DTXSID10590543 | |
Description | DSSTox provides a high quality public chemistry resource for supporting improved predictive toxicology. | |
Disclaimer and Information on In-Vitro Research Products
Please be aware that all articles and product information presented on BenchChem are intended solely for informational purposes. The products available for purchase on BenchChem are specifically designed for in-vitro studies, which are conducted outside of living organisms. In-vitro studies, derived from the Latin term "in glass," involve experiments performed in controlled laboratory settings using cells or tissues. It is important to note that these products are not categorized as medicines or drugs, and they have not received approval from the FDA for the prevention, treatment, or cure of any medical condition, ailment, or disease. We must emphasize that any form of bodily introduction of these products into humans or animals is strictly prohibited by law. It is essential to adhere to these guidelines to ensure compliance with legal and ethical standards in research and experimentation.