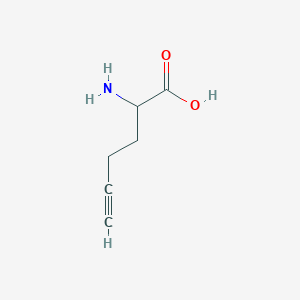
2-Amino-5-hexynoic acid
Overview
Description
Homopropargylglycine is a non-canonical amino acid analog of methionine. It contains an alkyne moiety, which allows it to be incorporated into proteins during active protein synthesis. This compound is particularly useful in bio-orthogonal chemistry, where it facilitates the tagging and visualization of newly synthesized proteins through click chemistry reactions .
Preparation Methods
Synthetic Routes and Reaction Conditions: Homopropargylglycine can be synthesized through various methods. One common approach involves the reaction of propargyl bromide with glycine in the presence of a base, such as sodium hydroxide, to form the desired product. The reaction typically occurs under mild conditions and can be completed within a few hours .
Industrial Production Methods: In industrial settings, homopropargylglycine is often produced using automated peptide synthesizers. These machines allow for the precise control of reaction conditions and the efficient production of large quantities of the compound. The use of solid-phase peptide synthesis techniques further enhances the yield and purity of the final product .
Chemical Reactions Analysis
Types of Reactions: Homopropargylglycine undergoes various chemical reactions, including:
Oxidation: The alkyne moiety can be oxidized to form a variety of products, depending on the specific reagents and conditions used.
Reduction: The alkyne group can be reduced to an alkene or alkane using hydrogenation reactions.
Common Reagents and Conditions:
Oxidation: Potassium permanganate or osmium tetroxide can be used as oxidizing agents.
Reduction: Palladium on carbon (Pd/C) with hydrogen gas is commonly used for hydrogenation reactions.
Substitution: Copper-catalyzed azide-alkyne cycloaddition (CuAAC) is a widely used reaction for incorporating homopropargylglycine into proteins.
Major Products: The major products formed from these reactions include various oxidized, reduced, and substituted derivatives of homopropargylglycine, which can be further utilized in biochemical and pharmaceutical research .
Scientific Research Applications
Homopropargylglycine has numerous applications in scientific research:
Chemistry: It is used in click chemistry for the selective tagging and visualization of newly synthesized proteins.
Biology: Homopropargylglycine is employed in studies of protein synthesis and turnover, allowing researchers to track the incorporation of new proteins in living cells.
Medicine: The compound is used in drug discovery and development, particularly in the design of novel therapeutics that target specific proteins.
Industry: Homopropargylglycine is utilized in the production of biopharmaceuticals and other high-value protein products
Mechanism of Action
Homopropargylglycine exerts its effects by being incorporated into proteins in place of methionine during translation. The alkyne moiety allows for subsequent tagging with azide-containing probes through click chemistry. This enables the selective visualization and analysis of newly synthesized proteins. The molecular targets and pathways involved include the ribosomal machinery responsible for protein synthesis and the various enzymes that facilitate click chemistry reactions .
Comparison with Similar Compounds
Homopropargylglycine is unique among non-canonical amino acids due to its alkyne moiety, which allows for efficient click chemistry reactions. Similar compounds include:
Azidohomoalanine: Contains an azide group instead of an alkyne, used for similar purposes in bio-orthogonal chemistry.
Photo-methionine: A photoreactive analog of methionine used for studying protein-protein interactions.
L-azidohomoalanine: Another methionine analog with an azide group, used for protein labeling and visualization
Homopropargylglycine’s unique alkyne functionality makes it particularly suitable for applications requiring high specificity and sensitivity in protein tagging and visualization.
Properties
IUPAC Name |
2-aminohex-5-ynoic acid | |
---|---|---|
Details | Computed by LexiChem 2.6.6 (PubChem release 2019.06.18) | |
Source | PubChem | |
URL | https://pubchem.ncbi.nlm.nih.gov | |
Description | Data deposited in or computed by PubChem | |
InChI |
InChI=1S/C6H9NO2/c1-2-3-4-5(7)6(8)9/h1,5H,3-4,7H2,(H,8,9) | |
Details | Computed by InChI 1.0.5 (PubChem release 2019.06.18) | |
Source | PubChem | |
URL | https://pubchem.ncbi.nlm.nih.gov | |
Description | Data deposited in or computed by PubChem | |
InChI Key |
SCGJGNWMYSYORS-UHFFFAOYSA-N | |
Details | Computed by InChI 1.0.5 (PubChem release 2019.06.18) | |
Source | PubChem | |
URL | https://pubchem.ncbi.nlm.nih.gov | |
Description | Data deposited in or computed by PubChem | |
Canonical SMILES |
C#CCCC(C(=O)O)N | |
Details | Computed by OEChem 2.1.5 (PubChem release 2019.06.18) | |
Source | PubChem | |
URL | https://pubchem.ncbi.nlm.nih.gov | |
Description | Data deposited in or computed by PubChem | |
Molecular Formula |
C6H9NO2 | |
Details | Computed by PubChem 2.1 (PubChem release 2019.06.18) | |
Source | PubChem | |
URL | https://pubchem.ncbi.nlm.nih.gov | |
Description | Data deposited in or computed by PubChem | |
Molecular Weight |
127.14 g/mol | |
Details | Computed by PubChem 2.1 (PubChem release 2021.05.07) | |
Source | PubChem | |
URL | https://pubchem.ncbi.nlm.nih.gov | |
Description | Data deposited in or computed by PubChem | |
Retrosynthesis Analysis
AI-Powered Synthesis Planning: Our tool employs the Template_relevance Pistachio, Template_relevance Bkms_metabolic, Template_relevance Pistachio_ringbreaker, Template_relevance Reaxys, Template_relevance Reaxys_biocatalysis model, leveraging a vast database of chemical reactions to predict feasible synthetic routes.
One-Step Synthesis Focus: Specifically designed for one-step synthesis, it provides concise and direct routes for your target compounds, streamlining the synthesis process.
Accurate Predictions: Utilizing the extensive PISTACHIO, BKMS_METABOLIC, PISTACHIO_RINGBREAKER, REAXYS, REAXYS_BIOCATALYSIS database, our tool offers high-accuracy predictions, reflecting the latest in chemical research and data.
Strategy Settings
Precursor scoring | Relevance Heuristic |
---|---|
Min. plausibility | 0.01 |
Model | Template_relevance |
Template Set | Pistachio/Bkms_metabolic/Pistachio_ringbreaker/Reaxys/Reaxys_biocatalysis |
Top-N result to add to graph | 6 |
Feasible Synthetic Routes
Disclaimer and Information on In-Vitro Research Products
Please be aware that all articles and product information presented on BenchChem are intended solely for informational purposes. The products available for purchase on BenchChem are specifically designed for in-vitro studies, which are conducted outside of living organisms. In-vitro studies, derived from the Latin term "in glass," involve experiments performed in controlled laboratory settings using cells or tissues. It is important to note that these products are not categorized as medicines or drugs, and they have not received approval from the FDA for the prevention, treatment, or cure of any medical condition, ailment, or disease. We must emphasize that any form of bodily introduction of these products into humans or animals is strictly prohibited by law. It is essential to adhere to these guidelines to ensure compliance with legal and ethical standards in research and experimentation.