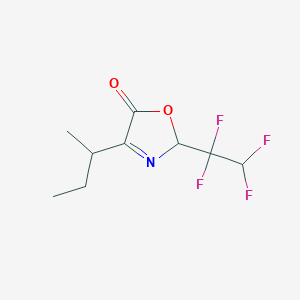
5(2H)-Oxazolone, 4-(1-methylpropyl)-2-(1,1,2,2-tetrafluoroethyl)-
- Click on QUICK INQUIRY to receive a quote from our team of experts.
- With the quality product at a COMPETITIVE price, you can focus more on your research.
Overview
Description
4-(sec-Butyl)-2-(1,1,2,2-tetrafluoroethyl)oxazol-5(2H)-one is an organic compound belonging to the oxazolone family Oxazolones are known for their diverse applications in organic synthesis, pharmaceuticals, and materials science
Preparation Methods
Synthetic Routes and Reaction Conditions
The synthesis of 4-(sec-Butyl)-2-(1,1,2,2-tetrafluoroethyl)oxazol-5(2H)-one typically involves the cyclization of appropriate precursors under controlled conditions. One possible route could be the reaction of a sec-butyl-substituted amine with a tetrafluoroethyl-substituted carboxylic acid derivative, followed by cyclization to form the oxazolone ring.
Industrial Production Methods
Industrial production methods for such compounds often involve optimized reaction conditions to maximize yield and purity. This may include the use of catalysts, specific solvents, and temperature control to ensure efficient synthesis.
Chemical Reactions Analysis
Types of Reactions
4-(sec-Butyl)-2-(1,1,2,2-tetrafluoroethyl)oxazol-5(2H)-one can undergo various chemical reactions, including:
Oxidation: The compound may be oxidized to form corresponding oxazolone derivatives with different oxidation states.
Reduction: Reduction reactions can lead to the formation of reduced oxazolone derivatives.
Substitution: The compound can participate in substitution reactions, where one or more substituents are replaced by other functional groups.
Common Reagents and Conditions
Common reagents for these reactions include oxidizing agents (e.g., potassium permanganate), reducing agents (e.g., lithium aluminum hydride), and nucleophiles for substitution reactions. Reaction conditions such as temperature, solvent, and pH play a crucial role in determining the outcome of these reactions.
Major Products Formed
The major products formed from these reactions depend on the specific reagents and conditions used. For example, oxidation may yield oxazolone derivatives with higher oxidation states, while substitution reactions can introduce new functional groups into the molecule.
Scientific Research Applications
Chemistry
In chemistry, 4-(sec-Butyl)-2-(1,1,2,2-tetrafluoroethyl)oxazol-5(2H)-one can be used as a building block for the synthesis of more complex molecules. Its unique structure allows for the exploration of new synthetic pathways and the development of novel compounds.
Biology
In biological research, oxazolones are often studied for their potential biological activities. This compound may exhibit interesting interactions with biological molecules, making it a candidate for further investigation in drug discovery and development.
Medicine
In medicine, oxazolone derivatives have been explored for their potential therapeutic properties. This compound could be investigated for its potential as a pharmaceutical agent, particularly in areas such as anti-inflammatory or antimicrobial research.
Industry
In industry, 4-(sec-Butyl)-2-(1,1,2,2-tetrafluoroethyl)oxazol-5(2H)-one may find applications in the development of new materials, such as polymers or coatings, due to its unique chemical properties.
Mechanism of Action
The mechanism of action of 4-(sec-Butyl)-2-(1,1,2,2-tetrafluoroethyl)oxazol-5(2H)-one would depend on its specific interactions with molecular targets. This could involve binding to enzymes or receptors, modulating their activity, and influencing various biochemical pathways. Detailed studies would be required to elucidate the exact mechanism and molecular targets involved.
Comparison with Similar Compounds
Similar Compounds
Similar compounds to 4-(sec-Butyl)-2-(1,1,2,2-tetrafluoroethyl)oxazol-5(2H)-one include other oxazolone derivatives with different substituents. Examples include:
- 4-(sec-Butyl)-2-(1,1,2,2-tetrafluoroethyl)oxazol-5(2H)-one
- 4-(tert-Butyl)-2-(1,1,2,2-tetrafluoroethyl)oxazol-5(2H)-one
- 4-(sec-Butyl)-2-(1,1,2,2-tetrafluoroethyl)oxazol-5(2H)-one
Uniqueness
The uniqueness of 4-(sec-Butyl)-2-(1,1,2,2-tetrafluoroethyl)oxazol-5(2H)-one lies in its specific substituents, which can influence its chemical reactivity, physical properties, and potential applications. The presence of the tetrafluoroethyl group, in particular, may impart unique characteristics compared to other oxazolone derivatives.
Properties
CAS No. |
51314-52-4 |
---|---|
Molecular Formula |
C9H11F4NO2 |
Molecular Weight |
241.18 g/mol |
IUPAC Name |
4-butan-2-yl-2-(1,1,2,2-tetrafluoroethyl)-2H-1,3-oxazol-5-one |
InChI |
InChI=1S/C9H11F4NO2/c1-3-4(2)5-6(15)16-8(14-5)9(12,13)7(10)11/h4,7-8H,3H2,1-2H3 |
InChI Key |
FWIZMVLAGVJNGT-UHFFFAOYSA-N |
Canonical SMILES |
CCC(C)C1=NC(OC1=O)C(C(F)F)(F)F |
Origin of Product |
United States |
Disclaimer and Information on In-Vitro Research Products
Please be aware that all articles and product information presented on BenchChem are intended solely for informational purposes. The products available for purchase on BenchChem are specifically designed for in-vitro studies, which are conducted outside of living organisms. In-vitro studies, derived from the Latin term "in glass," involve experiments performed in controlled laboratory settings using cells or tissues. It is important to note that these products are not categorized as medicines or drugs, and they have not received approval from the FDA for the prevention, treatment, or cure of any medical condition, ailment, or disease. We must emphasize that any form of bodily introduction of these products into humans or animals is strictly prohibited by law. It is essential to adhere to these guidelines to ensure compliance with legal and ethical standards in research and experimentation.