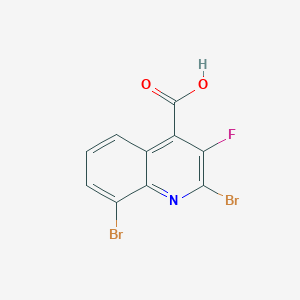
4-Quinolinecarboxylic acid, 2,8-dibromo-3-fluoro-
- Click on QUICK INQUIRY to receive a quote from our team of experts.
- With the quality product at a COMPETITIVE price, you can focus more on your research.
Overview
Description
Preparation Methods
Synthetic Routes and Reaction Conditions
The synthesis of 2,8-dibromo-3-fluoroquinoline-4-carboxylic acid typically involves multi-step reactions starting from readily available precursors. One common method includes the bromination of 3-fluoroquinoline-4-carboxylic acid, followed by further functionalization to introduce the second bromine atom at the 8-position . The reaction conditions often involve the use of brominating agents such as N-bromosuccinimide (NBS) in the presence of a catalyst like iron(III) bromide (FeBr3) under controlled temperature and solvent conditions .
Industrial Production Methods
Industrial production of this compound may involve similar synthetic routes but on a larger scale, with optimizations for yield and purity. The use of continuous flow reactors and automated systems can enhance the efficiency and scalability of the production process .
Chemical Reactions Analysis
Types of Reactions
2,8-Dibromo-3-fluoroquinoline-4-carboxylic acid undergoes various types of chemical reactions, including:
Substitution Reactions: The bromine atoms can be substituted with other functional groups through nucleophilic substitution reactions.
Oxidation and Reduction: The quinoline ring can undergo oxidation and reduction reactions, altering its electronic properties.
Cross-Coupling Reactions: The compound can participate in cross-coupling reactions, such as Suzuki-Miyaura coupling, to form more complex molecules.
Common Reagents and Conditions
Common reagents used in these reactions include palladium catalysts for cross-coupling reactions, oxidizing agents like potassium permanganate for oxidation, and reducing agents such as sodium borohydride for reduction . Reaction conditions vary depending on the desired transformation but typically involve controlled temperatures and inert atmospheres to prevent unwanted side reactions .
Major Products Formed
The major products formed from these reactions depend on the specific reagents and conditions used. For example, nucleophilic substitution can yield derivatives with different functional groups, while cross-coupling reactions can produce biaryl compounds .
Scientific Research Applications
Antibacterial Properties
One of the primary applications of 4-Quinolinecarboxylic acid derivatives is their antibacterial activity. Research indicates that quinoline and naphthyridine carboxylic acid derivatives exhibit significant antibacterial properties against a range of infectious diseases. The structure of these compounds allows for modifications that enhance their efficacy as antibiotics. For instance, compounds with a carbon-nitrogen linkage at specific positions have demonstrated improved antibacterial activity .
Case Study:
A study highlighted the synthesis of various quinoline derivatives, including those based on 4-Quinolinecarboxylic acid, which were evaluated for their antibacterial properties. The results showed that specific modifications led to enhanced activity against Gram-positive and Gram-negative bacteria .
Antiviral Activity
Beyond antibacterial applications, quinoline derivatives have also been investigated for their antiviral properties. Compounds such as 8-hydroxy-7-substituted quinolines have shown promise as antiviral agents, with potential applications in treating viral infections. The structural features of these compounds contribute to their mechanism of action against viruses .
Case Study:
Research into the antiviral activity of quinoline derivatives indicated that specific modifications can lead to increased efficacy against viral pathogens. This opens avenues for developing new antiviral therapies based on the quinoline framework .
Antimalarial Potential
Quinolines are well-known for their antimalarial potential, particularly in the treatment of malaria caused by Plasmodium species. The derivatives of 4-Quinolinecarboxylic acid may contribute to this field by offering new compounds with enhanced potency and reduced side effects compared to traditional antimalarial drugs .
Case Study:
A synthesis study reported novel fluoroquinoline derivatives that exhibited significant antimalarial activity in vitro. These findings suggest that further exploration of 4-Quinolinecarboxylic acid derivatives could lead to effective treatments for malaria .
Antitumor Activity
Another promising application of 4-Quinolinecarboxylic acid derivatives is in cancer research. Certain quinoline derivatives have been shown to cap the N-terminal of natural cyclic peptides with antitumoral activity, suggesting potential use in cancer therapeutics .
Case Study:
Research focusing on quinoline derivatives demonstrated their ability to inhibit tumor growth in various cancer cell lines. The study emphasized the importance of structural modifications in enhancing the antitumor efficacy of these compounds .
Chemical Synthesis and Modifications
The synthesis and modification of 4-Quinolinecarboxylic acid are critical for expanding its applications. Various synthetic routes have been explored to produce derivatives with improved biological activities or novel properties.
Synthesis Overview:
A four-step synthetic procedure involving protection and oxidation steps has been reported for preparing 3-hydroxyquinoline-2-carboxylic acid from simpler precursors. This method allows for the introduction of various substituents that can enhance biological activity .
Data Table: Applications Overview
Application Area | Description | Key Findings |
---|---|---|
Antibacterial | Effective against Gram-positive and Gram-negative bacteria | Enhanced activity through structural modifications |
Antiviral | Potential against viral infections | Increased efficacy with specific modifications |
Antimalarial | Treatment for malaria | Significant activity observed in vitro |
Antitumor | Inhibitory effects on tumor growth | Structural modifications enhance efficacy |
Chemical Synthesis | Routes for producing active derivatives | Four-step synthesis yielding various derivatives |
Mechanism of Action
The mechanism of action of 2,8-dibromo-3-fluoroquinoline-4-carboxylic acid involves its interaction with specific molecular targets, such as bacterial enzymes DNA gyrase and DNA topoisomerase IV . By stabilizing the enzyme-DNA complex and causing DNA cleavage, the compound effectively inhibits bacterial growth and proliferation . This mechanism is similar to that of other fluoroquinolones, which are known for their broad-spectrum antibacterial activity .
Comparison with Similar Compounds
Similar Compounds
- 2,8-Dibromoquinoline-4-carboxylic acid
- 3-Fluoroquinoline-4-carboxylic acid
- 2,8-Dichloro-3-fluoroquinoline-4-carboxylic acid
Uniqueness
2,8-Dibromo-3-fluoroquinoline-4-carboxylic acid is unique due to the presence of both bromine and fluorine atoms, which impart distinct electronic and steric properties.
Biological Activity
4-Quinolinecarboxylic acid, 2,8-dibromo-3-fluoro- (chemical formula: C10H4Br2FNO2) is a derivative of quinoline known for its diverse biological activities. Quinoline and its derivatives have garnered significant attention in medicinal chemistry due to their potential therapeutic applications, including antimicrobial, anticancer, and anti-inflammatory properties. This article delves into the biological activity of this specific compound, highlighting key research findings, mechanisms of action, and potential applications.
Property | Value |
---|---|
Molecular Formula | C10H4Br2FNO2 |
Molecular Weight | 319.95 g/mol |
IUPAC Name | 2,8-Dibromo-3-fluoro-4-quinolinecarboxylic acid |
InChI Key | DQFQZLZJZKATMG-UHFFFAOYSA-N |
Antimicrobial Properties
Research indicates that quinoline derivatives exhibit significant antimicrobial activity. A study on related quinoline compounds demonstrated moderate antibacterial effects against a variety of Gram-positive and Gram-negative bacteria. While specific data on 4-Quinolinecarboxylic acid, 2,8-dibromo-3-fluoro- is limited, its structural similarities suggest potential efficacy against bacterial strains such as Staphylococcus aureus and Escherichia coli .
Anticancer Activity
The anticancer potential of quinoline derivatives has been well-documented. For instance, compounds structurally similar to 4-Quinolinecarboxylic acid have shown promising results in inhibiting cancer cell proliferation. In vitro studies have indicated that certain quinoline derivatives can induce apoptosis in cancer cells by targeting specific pathways involved in cell cycle regulation and apoptosis .
In a notable study focusing on SIRT3 inhibitors, a series of quinoline derivatives were synthesized and evaluated for their antiproliferative activity against various cancer cell lines. The results indicated that modifications at the carboxylic acid position significantly enhanced the inhibitory potency against leukemia cells .
The biological activity of 4-Quinolinecarboxylic acid, 2,8-dibromo-3-fluoro- is likely mediated through several mechanisms:
- Enzyme Inhibition : Similar compounds have been shown to inhibit enzymes involved in critical cellular processes such as DNA replication and repair.
- Cell Cycle Arrest : Studies suggest that quinoline derivatives can induce cell cycle arrest at the G1 phase, leading to reduced proliferation of cancer cells .
- Apoptosis Induction : The ability to induce apoptosis in cancer cells is a key feature of many quinoline derivatives, attributed to their interaction with mitochondrial pathways .
Case Studies
- SIRT3 Inhibition : A study synthesized various quinoline derivatives and found that certain modifications led to enhanced SIRT3 inhibitory activity. Molecule P6 from this series exhibited a significant inhibitory rate against leukemic cell lines .
- Antimicrobial Evaluation : Various synthesized quinoline compounds were assessed for antibacterial activity using standard methods against multiple bacterial strains. The results indicated varying degrees of effectiveness, with some compounds showing particularly strong activity against Staphylococcus aureus .
Properties
CAS No. |
834884-17-2 |
---|---|
Molecular Formula |
C10H4Br2FNO2 |
Molecular Weight |
348.95 g/mol |
IUPAC Name |
2,8-dibromo-3-fluoroquinoline-4-carboxylic acid |
InChI |
InChI=1S/C10H4Br2FNO2/c11-5-3-1-2-4-6(10(15)16)7(13)9(12)14-8(4)5/h1-3H,(H,15,16) |
InChI Key |
XAOKHKOSPZABFP-UHFFFAOYSA-N |
Canonical SMILES |
C1=CC2=C(C(=C(N=C2C(=C1)Br)Br)F)C(=O)O |
Origin of Product |
United States |
Disclaimer and Information on In-Vitro Research Products
Please be aware that all articles and product information presented on BenchChem are intended solely for informational purposes. The products available for purchase on BenchChem are specifically designed for in-vitro studies, which are conducted outside of living organisms. In-vitro studies, derived from the Latin term "in glass," involve experiments performed in controlled laboratory settings using cells or tissues. It is important to note that these products are not categorized as medicines or drugs, and they have not received approval from the FDA for the prevention, treatment, or cure of any medical condition, ailment, or disease. We must emphasize that any form of bodily introduction of these products into humans or animals is strictly prohibited by law. It is essential to adhere to these guidelines to ensure compliance with legal and ethical standards in research and experimentation.