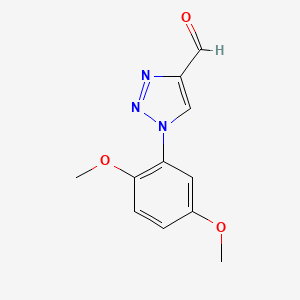
1-(2,5-dimethoxyphenyl)-1H-1,2,3-triazole-4-carbaldehyde
- Click on QUICK INQUIRY to receive a quote from our team of experts.
- With the quality product at a COMPETITIVE price, you can focus more on your research.
Overview
Description
1-(2,5-Dimethoxyphenyl)-1H-1,2,3-triazole-4-carbaldehyde is a heterocyclic compound featuring a triazole core substituted with a 2,5-dimethoxyphenyl group and a carbaldehyde functional group. The triazole ring is a versatile scaffold in medicinal and materials chemistry due to its stability and capacity for hydrogen bonding. The 2,5-dimethoxyphenyl substituent introduces electron-donating methoxy groups at the meta and para positions of the aromatic ring, which can influence electronic properties, solubility, and intermolecular interactions.
Preparation Methods
The synthesis of 1-(2,5-dimethoxyphenyl)-1H-1,2,3-triazole-4-carbaldehyde typically involves a multi-step process. One common method starts with the preparation of 2,5-dimethoxybenzaldehyde, which is then subjected to a series of reactions to introduce the triazole ring and the aldehyde group. The key steps in the synthesis include:
Formation of 2,5-dimethoxybenzaldehyde: This can be achieved through the oxidation of 2,5-dimethoxytoluene using oxidizing agents such as potassium permanganate or chromium trioxide.
Cycloaddition Reaction: The 2,5-dimethoxybenzaldehyde is then reacted with an azide compound in the presence of a copper catalyst to form the triazole ring through a Huisgen cycloaddition reaction.
Introduction of the Aldehyde Group:
Industrial production methods for this compound would likely involve optimization of these steps to ensure high yield and purity, as well as scalability for large-scale synthesis.
Chemical Reactions Analysis
Nucleophilic Addition Reactions
The aldehyde group readily participates in nucleophilic additions. For example:
-
Schiff Base Formation : Reacts with primary amines to form imines.
-
Cyanohydrin Synthesis :
Oxidation
-
Carboxylic Acid Formation :
-
Reagents : KMnO₄ in acidic or alkaline conditions.
-
Yield : ~85% under optimized conditions.
-
Reduction
Cyclization Reactions
The compound serves as a precursor for heterocyclic systems:
-
Imidazolidine Formation :
-
Thiazole Synthesis :
Cross-Coupling Reactions
The triazole ring directs regioselective coupling:
-
Suzuki–Miyaura Coupling :
Condensation Reactions
-
Aldol Condensation :
-
Perkin Reaction :
Electrophilic Substitution
The electron-rich triazole ring undergoes halogenation:
-
Bromination :
-
Reagents : Br₂ in CCl₄, FeCl₃ catalyst.
-
Regioselectivity : Bromination at C5 of the triazole.
-
Multi-Component Reactions (MCRs)
-
Triazole-Alkyne Cycloaddition :
Reaction Optimization for Imine Formation
Amine | Solvent | Temp (°C) | Time (h) | Yield (%) |
---|---|---|---|---|
4-Aminophenol | Ethanol | 60 | 6 | 88 |
Benzylamine | THF | 25 | 12 | 72 |
Biological Activity of Derivatives
Derivative | IC₅₀ (µM) | Target |
---|---|---|
Schiff Base (4-NO₂) | 1.2 | EGFR Kinase |
Thiazole Hybrid | 4.8 | E. coli |
Scientific Research Applications
Synthesis and Characterization
The synthesis of 1-(2,5-dimethoxyphenyl)-1H-1,2,3-triazole-4-carbaldehyde typically involves the reaction of diazomalonaldehyde with appropriate aniline hydrochlorides. This process yields various N-substituted phenyl-1,2,3-triazole-4-carbaldehydes. The compound has been characterized using techniques such as NMR spectroscopy and X-ray crystallography, confirming its structural integrity and purity .
Antimicrobial Properties
Research indicates that derivatives of this compound exhibit antimicrobial activity against both Gram-positive and Gram-negative bacteria. For instance, studies have shown that certain triazole derivatives possess minimum inhibitory concentrations (MICs) ranging from 0.10 µg/mL to 100 µg/mL against various bacterial strains .
Antitubercular Activity
The compound has demonstrated promising antitubercular activity in vitro. A study evaluated the structure-activity relationship (SAR) of several triazole derivatives, revealing that modifications to the triazole ring can enhance their efficacy against Mycobacterium tuberculosis. Specifically, derivatives with fluorinated groups showed increased activity compared to their non-fluorinated counterparts .
Anticancer Potential
Recent investigations into the anticancer properties of this compound have yielded encouraging results. The compound has been found to induce cell cycle arrest in various cancer cell lines by modulating cyclin-dependent kinases (CDKs). For example, it was observed to inhibit CDK4 and cyclin D1 expression in human carcinoma cells .
Case Studies
Structure-Activity Relationship (SAR)
The SAR studies conducted on this compound indicate that modifications to the phenyl ring and the triazole moiety significantly influence biological activity. For instance:
Mechanism of Action
The mechanism of action of 1-(2,5-dimethoxyphenyl)-1H-1,2,3-triazole-4-carbaldehyde is not fully understood, but it is believed to involve interactions with specific molecular targets and pathways. The triazole ring can interact with enzymes and receptors, potentially inhibiting their activity or modulating their function. The methoxy groups and aldehyde functional group may also contribute to the compound’s biological activity by influencing its binding affinity and specificity.
Comparison with Similar Compounds
Comparison with Structurally Similar Compounds
Table 1: Structural and Functional Comparison of Triazole Derivatives
*Calculated molecular weight based on formula C₁₁H₁₁N₃O₃.
Substituent Effects on Physicochemical Properties
- Electron-Donating vs. Electron-Withdrawing Groups: The 2,5-dimethoxy groups (target compound) are strongly electron-donating, enhancing resonance stabilization of the phenyl ring and increasing polarity compared to 4-fluoro (electron-withdrawing) or 4-methoxy (single electron-donating) analogs .
Molecular Weight and Solubility :
Pharmacological and Functional Potential
- Bioactivity: Methoxy groups are associated with enhanced interaction with biological targets (e.g., enzymes, receptors) due to hydrogen-bonding capacity. The dual methoxy substitution in the target compound may offer superior binding affinity compared to monosubstituted analogs . Carbaldehyde Reactivity: The aldehyde group enables facile derivatization (e.g., Schiff base formation), making the compound a valuable intermediate for drug discovery. In contrast, the carboxylic acid derivative () is more suited for salt formation or esterification .
Lab Applications :
- The 4-fluoro analog () is marketed as a "versatile small molecule scaffold," highlighting the role of triazole-carbaldehydes in modular synthesis. The target compound’s dimethoxy substitution could expand its utility in designing fluorescence probes or metal-organic frameworks .
Biological Activity
1-(2,5-Dimethoxyphenyl)-1H-1,2,3-triazole-4-carbaldehyde is a compound of interest in medicinal chemistry due to its potential biological activities. This article reviews the synthesis, biological evaluation, and mechanisms of action associated with this compound, drawing from diverse sources to provide a comprehensive overview.
Synthesis
The synthesis of this compound typically involves the reaction of 2,5-dimethoxyphenyl azide with appropriate alkyne derivatives under copper-catalyzed conditions. The resulting triazole can be further modified to introduce the aldehyde functional group. The purity and structure of the synthesized compound are confirmed through techniques such as NMR spectroscopy and mass spectrometry.
Anticancer Properties
Recent studies have highlighted the anticancer potential of triazole derivatives. For instance, compounds containing the triazole ring have shown significant antiproliferative effects against various cancer cell lines. Table 1 summarizes the IC50 values of related triazole compounds against different cancer types:
The mechanism of action for these compounds often involves the induction of apoptosis through mitochondrial pathways and the inhibition of cell cycle progression.
Antimicrobial Activity
In addition to anticancer effects, triazole derivatives have exhibited antimicrobial activity . For example, certain triazoles have demonstrated effectiveness against Escherichia coli and Staphylococcus aureus. The antimicrobial efficacy is attributed to their ability to disrupt microbial cell membranes or inhibit essential metabolic pathways.
Case Studies
A notable study investigated a series of triazole derivatives similar to this compound. These compounds were tested for their cytotoxicity against leukemia cell lines (SR, MOLT-4) and showed promising results comparable to established chemotherapeutics like doxorubicin .
Another study focused on the structure-activity relationship (SAR) of various substituted triazoles. It was found that modifications at the phenyl ring significantly influenced biological activity, with specific substituents enhancing potency against cancer cells .
The biological activity of this compound can be attributed to several mechanisms:
- Apoptosis Induction : Many triazoles trigger apoptotic pathways by increasing reactive oxygen species (ROS) levels and activating caspases.
- Cell Cycle Arrest : Compounds can induce cell cycle arrest at various phases, particularly G0/G1 and G2/M phases.
- Microtubule Disruption : Some derivatives act as microtubule-destabilizing agents, which is critical in cancer treatment strategies.
Q & A
Q. What are the common synthetic routes for 1-(2,5-dimethoxyphenyl)-1H-1,2,3-triazole-4-carbaldehyde?
Basic
The compound is synthesized via two primary approaches:
- Click Chemistry Followed by Oxidation : A triazole alcohol intermediate is first formed via copper-catalyzed azide-alkyne cycloaddition (CuAAC), then oxidized to the carbaldehyde using Jones reagent (CrO₃ in H₂SO₄). This method is scalable and yields high-purity products .
- One-Pot Multicomponent Reactions : The Hantzsch dihydropyridine reaction combines 1-(2-nitrophenyl)-1H-1,2,3-triazole-4-carbaldehyde, ethyl acetoacetate, and ammonium acetate in ethanol at 80°C for 2 hours, achieving 85% yield after crystallization .
Advanced
Alternative routes include:
- Vilsmeier-Haack Reaction : Applied to acetophenone phenylhydrazones, this method introduces formyl groups via POCl₃ and DMF, though yields for triazole derivatives require optimization .
- Oxidative Strategies : Comparative studies between Jones reagent and milder oxidants (e.g., TEMPO/NaClO) could improve selectivity and reduce side reactions in triazole oxidation .
Q. How is the compound purified after synthesis?
Basic
- Crystallization : Ethanol is commonly used for recrystallization due to the compound’s moderate solubility, yielding high-purity crystals (e.g., 85% recovery in Hantzsch reactions) .
- Vacuum Filtration : Post-reflux, the crude product is isolated via filtration after solvent evaporation under reduced pressure .
Advanced
- Chromatographic Techniques : While not explicitly detailed in the evidence, silica gel chromatography (using ethyl acetate/hexane gradients) is inferred for resolving byproducts in multicomponent reactions .
Q. What spectroscopic methods are used to characterize the compound?
Basic
- NMR Spectroscopy : ¹H and ¹³C NMR confirm the triazole core and aldehyde proton (δ ~9.8–10.2 ppm). Aromatic protons from the 2,5-dimethoxyphenyl group appear as distinct doublets .
- Mass Spectrometry (MS) : High-resolution MS validates the molecular ion peak (e.g., m/z 273.1 for C₁₂H₁₁N₃O₃) .
Advanced
- X-Ray Crystallography : SHELXL refines crystal structures, resolving anisotropic displacement parameters. WinGX/ORTEP visualizes molecular packing and hydrogen-bonding networks .
Q. How can reaction conditions be optimized to improve yield?
Advanced
- Temperature and Catalyst Screening : Higher temperatures (80–100°C) in ethanol enhance Hantzsch reaction kinetics, while Cu(I) catalysts (e.g., CuI vs. CuSO₄/ascorbate) may improve CuAAC efficiency .
- Solvent Effects : Polar aprotic solvents (e.g., DMF) could accelerate Vilsmeier-Haack formylation but may require stringent drying to avoid hydrolysis .
Q. How to address discrepancies between experimental and theoretical spectroscopic data?
Advanced
- 2D NMR (HSQC, HMBC) : Resolves ambiguous ¹H-¹³C correlations, particularly for overlapping aromatic signals .
- Computational Validation : Density Functional Theory (DFT) predicts NMR chemical shifts, identifying misassignments (e.g., aldehyde vs. ketone resonances) .
Q. What computational methods assist in studying the compound’s reactivity?
Advanced
- Docking Studies : Molecular docking (AutoDock Vina) evaluates interactions with biological targets (e.g., cholinesterase active sites), guiding derivative design .
- DFT Calculations : Models frontier molecular orbitals to predict regioselectivity in electrophilic substitutions .
Q. How to design derivatives for enhanced biological activity?
Advanced
- Structure-Activity Relationship (SAR) : Substituent effects are tested by modifying the dimethoxyphenyl group (e.g., electron-withdrawing nitro or bromo groups) or triazole position .
- Bioisosteric Replacement : Replacing the aldehyde with carboxamide or hydroxymethyl groups improves solubility and target affinity .
Q. What strategies are effective in crystallizing the compound for X-ray analysis?
Advanced
- Slow Evaporation : Ethanol or methanol solutions are evaporated at 4°C to grow single crystals suitable for SHELXL refinement .
- Additive Screening : Small amounts of DMSO or acetic acid may stabilize crystal lattices by promoting hydrogen bonds .
Q. How to analyze byproducts formed during synthesis?
Advanced
- TLC Monitoring : Rf values (e.g., 0.3 in ethyl acetate/hexane) track reaction progress and byproduct formation .
- LC-MS : Identifies impurities via molecular ion peaks and fragmentation patterns, guiding column chromatography optimization .
Q. What are best practices for resolving crystal structures using software like SHELXL?
Advanced
Properties
CAS No. |
916151-02-5 |
---|---|
Molecular Formula |
C11H11N3O3 |
Molecular Weight |
233.22 g/mol |
IUPAC Name |
1-(2,5-dimethoxyphenyl)triazole-4-carbaldehyde |
InChI |
InChI=1S/C11H11N3O3/c1-16-9-3-4-11(17-2)10(5-9)14-6-8(7-15)12-13-14/h3-7H,1-2H3 |
InChI Key |
YZFKWAFEOTYBIE-UHFFFAOYSA-N |
Canonical SMILES |
COC1=CC(=C(C=C1)OC)N2C=C(N=N2)C=O |
Origin of Product |
United States |
Disclaimer and Information on In-Vitro Research Products
Please be aware that all articles and product information presented on BenchChem are intended solely for informational purposes. The products available for purchase on BenchChem are specifically designed for in-vitro studies, which are conducted outside of living organisms. In-vitro studies, derived from the Latin term "in glass," involve experiments performed in controlled laboratory settings using cells or tissues. It is important to note that these products are not categorized as medicines or drugs, and they have not received approval from the FDA for the prevention, treatment, or cure of any medical condition, ailment, or disease. We must emphasize that any form of bodily introduction of these products into humans or animals is strictly prohibited by law. It is essential to adhere to these guidelines to ensure compliance with legal and ethical standards in research and experimentation.