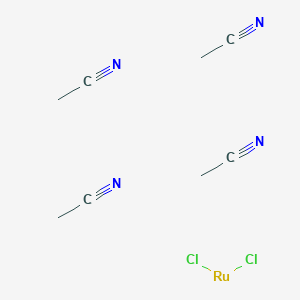
Dichlorotetrakis(acetonitrile)ruthenium
- Click on QUICK INQUIRY to receive a quote from our team of experts.
- With the quality product at a COMPETITIVE price, you can focus more on your research.
Overview
Description
Dichlorotetrakis(acetonitrile)ruthenium is a coordination compound with the formula [RuCl2(CH3CN)4]. This compound is of significant interest in the field of inorganic chemistry due to its versatile reactivity and potential applications in various scientific domains. Ruthenium, a transition metal, forms stable complexes with acetonitrile, making this compound a valuable precursor for synthesizing other ruthenium-based complexes.
Preparation Methods
Synthetic Routes and Reaction Conditions: Dichlorotetrakis(acetonitrile)ruthenium can be synthesized by reacting ruthenium trichloride (RuCl3) with acetonitrile (CH3CN) under specific conditions. The reaction typically involves dissolving ruthenium trichloride in acetonitrile and heating the mixture to facilitate the formation of the desired complex. The reaction can be represented as follows: [ \text{RuCl}_3 + 4 \text{CH}_3\text{CN} \rightarrow \text{[RuCl}_2(\text{CH}_3\text{CN})_4] ]
Industrial Production Methods: While the laboratory synthesis of this compound is well-documented, industrial production methods may involve scaling up the reaction using larger reactors and optimizing conditions to ensure high yield and purity. The use of automated systems and continuous flow reactors can enhance the efficiency of the production process.
Chemical Reactions Analysis
Types of Reactions: Dichlorotetrakis(acetonitrile)ruthenium undergoes various chemical reactions, including:
Substitution Reactions: The acetonitrile ligands can be replaced by other ligands, such as phosphines, amines, or other nitrogen-containing compounds.
Oxidation and Reduction Reactions: The ruthenium center can undergo oxidation or reduction, leading to the formation of different oxidation states and complexes.
Coordination Reactions: The compound can coordinate with other molecules or ions, forming new complexes with different properties.
Common Reagents and Conditions:
Substitution Reactions: Common reagents include phosphines (e.g., triphenylphosphine), amines (e.g., pyridine), and other ligands. These reactions are typically carried out in organic solvents such as dichloromethane or toluene.
Oxidation and Reduction Reactions: Reagents such as hydrogen peroxide (H2O2) or sodium borohydride (NaBH4) can be used to oxidize or reduce the ruthenium center.
Major Products Formed: The products formed from these reactions depend on the specific reagents and conditions used. For example, substitution reactions with phosphines can yield complexes like [RuCl2(PPh3)4], while oxidation reactions can produce higher oxidation state complexes.
Scientific Research Applications
Dichlorotetrakis(acetonitrile)ruthenium has a wide range of applications in scientific research, including:
Chemistry: It serves as a precursor for synthesizing various ruthenium complexes used in catalysis, materials science, and coordination chemistry.
Biology and Medicine: Ruthenium complexes, including those derived from this compound, have shown potential as anticancer agents due to their ability to interact with DNA and induce apoptosis in cancer cells.
Industry: The compound is used in the development of catalysts for chemical reactions, including hydrogenation, oxidation, and polymerization processes.
Mechanism of Action
The mechanism of action of dichlorotetrakis(acetonitrile)ruthenium and its derivatives involves coordination with target molecules, such as DNA or proteins. The ruthenium center can form covalent bonds with nucleophilic sites on these molecules, leading to structural changes and functional disruptions. In the case of anticancer activity, the compound can induce apoptosis by interacting with DNA and triggering cellular pathways that lead to programmed cell death.
Comparison with Similar Compounds
Dichlorotetrakis(dimethylsulfoxide)ruthenium(II): This compound has similar coordination properties but uses dimethylsulfoxide (DMSO) as ligands instead of acetonitrile.
Dichlorotetrakis(pyridine)ruthenium(II): This complex uses pyridine ligands and exhibits different reactivity and applications compared to the acetonitrile complex.
Uniqueness: Dichlorotetrakis(acetonitrile)ruthenium is unique due to its ability to easily undergo ligand substitution reactions, making it a versatile precursor for synthesizing a wide range of ruthenium complexes. Its stability and reactivity in various chemical environments also contribute to its distinctiveness.
Properties
CAS No. |
939024-67-6 |
---|---|
Molecular Formula |
C8H12Cl2N4Ru |
Molecular Weight |
336.2 g/mol |
IUPAC Name |
acetonitrile;dichlororuthenium |
InChI |
InChI=1S/4C2H3N.2ClH.Ru/c4*1-2-3;;;/h4*1H3;2*1H;/q;;;;;;+2/p-2 |
InChI Key |
SJRJYBLWSPYJOA-UHFFFAOYSA-L |
Canonical SMILES |
CC#N.CC#N.CC#N.CC#N.Cl[Ru]Cl |
Origin of Product |
United States |
Disclaimer and Information on In-Vitro Research Products
Please be aware that all articles and product information presented on BenchChem are intended solely for informational purposes. The products available for purchase on BenchChem are specifically designed for in-vitro studies, which are conducted outside of living organisms. In-vitro studies, derived from the Latin term "in glass," involve experiments performed in controlled laboratory settings using cells or tissues. It is important to note that these products are not categorized as medicines or drugs, and they have not received approval from the FDA for the prevention, treatment, or cure of any medical condition, ailment, or disease. We must emphasize that any form of bodily introduction of these products into humans or animals is strictly prohibited by law. It is essential to adhere to these guidelines to ensure compliance with legal and ethical standards in research and experimentation.