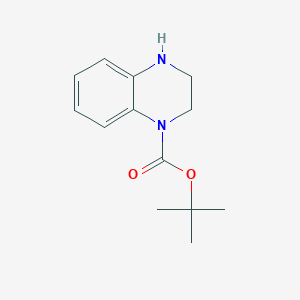
tert-Butyl 3,4-dihydroquinoxaline-1(2H)-carboxylate
Overview
Description
tert-Butyl 3,4-dihydroquinoxaline-1(2H)-carboxylate is a bicyclic heterocyclic compound featuring a quinoxaline core with a tert-butoxycarbonyl (Boc) protective group at the 1-position. This scaffold is widely utilized in medicinal chemistry and organic synthesis due to its versatility as a building block for pharmaceuticals, agrochemicals, and materials. The Boc group enhances stability and modulates solubility, making the compound suitable for diverse synthetic applications .
Preparation Methods
Synthetic Routes and Reaction Conditions
The synthesis of tert-Butyl 3,4-dihydroquinoxaline-1(2H)-carboxylate typically involves the reaction of 3,4-dihydroquinoxaline-1-carboxylic acid with tert-butyl alcohol in the presence of a dehydrating agent such as dicyclohexylcarbodiimide (DCC) and a catalyst like 4-dimethylaminopyridine (DMAP). The reaction is carried out under anhydrous conditions to prevent hydrolysis of the ester bond.
Industrial Production Methods
Industrial production methods for this compound are similar to laboratory synthesis but are scaled up to accommodate larger quantities. The process involves the use of continuous flow reactors to ensure efficient mixing and reaction control. The reaction conditions are optimized to maximize yield and purity while minimizing by-products.
Chemical Reactions Analysis
Esterification and Amidation
The tert-butyl ester group undergoes hydrolysis under acidic or basic conditions to yield the corresponding carboxylic acid, which can be further functionalized. For example:
- Esterification with alcohols (e.g., methanol, ethanol) in the presence of methanesulfonic acid supported on alumina produces derivatives with modified ester groups .
- Amidation reactions involve replacing the tert-butyl ester with amines. In one protocol, 2-cyanopiperidine-1-carbonyl chloride reacts with tert-butyl 3,4-dihydroquinoxaline-1(2H)-carboxylate to form carboxamide derivatives .
Example Reaction Conditions:
Nucleophilic Substitution
The quinoxaline core participates in nucleophilic substitution reactions. Brominated derivatives (e.g., at the 6-position) undergo substitution with nucleophiles such as amines or thiols:
- Bromine displacement by amines in polar aprotic solvents (e.g., DMF) yields amino-substituted quinoxalines.
Oxidation and Reduction
The dihydroquinoxaline ring system is redox-active:
- Oxidation with hydrogen peroxide converts the dihydroquinoxaline to a fully aromatic quinoxaline derivative .
- Reduction using catalytic hydrogenation (H₂/Pd-C) saturates the ring, forming tetrahydroquinoxaline analogs .
Cyclization and Ring Expansion
Under catalyst-free conditions, this compound reacts with tert-butyl isocyanide and ketones to form fused dihydroquinoxaline derivatives . For example:
- Reaction with acetone yields N-(3,3-dimethyl-3,4-dihydroquinoxalin-2(1H)-ylidene)-2-methylpropan-2-amine .
Comparative Reactivity
The tert-butyl group enhances steric hindrance, directing substitutions to specific positions. For example:
Position | Reactivity | Example Reaction |
---|---|---|
C-6 | High (due to bromine activation) | Nucleophilic substitution with amines |
C-3 | Moderate | Oxidation to ketone derivatives |
Scientific Research Applications
Antimicrobial Properties
Preliminary studies have indicated that tert-butyl 3,4-dihydroquinoxaline-1(2H)-carboxylate may exhibit antimicrobial properties. Its mechanism of action likely involves interaction with specific molecular targets such as enzymes and receptors, modulating their activity to inhibit microbial growth.
Case Study: Antimycobacterial Activity
Research has shown that structural modifications in quinoxaline derivatives can significantly influence their antimicrobial potency against pathogens like Mycobacterium tuberculosis. For example, compounds with bulky substituents have demonstrated enhanced activity against this bacterium.
Compound | MIC (μg/mL) | Activity Type |
---|---|---|
Compound A | 1.25 | Antimycobacterial |
Compound B | >10 | Less effective |
Compound C | 5 | Moderate activity |
Anticancer Properties
Quinoxaline derivatives are also recognized for their potential anticancer effects. The compound may induce apoptosis in cancer cell lines by activating caspase pathways, leading to cell death. This property is attributed to its ability to interact with various biological targets, modulating signaling pathways involved in cancer progression.
Case Study: Induction of Apoptosis
In a study examining quinoxaline derivatives, it was found that specific structural features were crucial in determining the compound's ability to trigger apoptosis effectively. The results suggest that this compound could be a promising candidate for further investigation in cancer therapy.
Mechanistic Insights
The biological activity of this compound is likely mediated through interactions with cytochrome P450 enzymes, which play a critical role in drug metabolism. This interaction could influence the pharmacokinetics and pharmacodynamics of therapeutic agents.
Mechanism of Action
The mechanism of action of tert-Butyl 3,4-dihydroquinoxaline-1(2H)-carboxylate involves its interaction with molecular targets such as enzymes and receptors. The compound can bind to active sites, inhibiting or modulating the activity of these targets. The specific pathways involved depend on the biological context and the nature of the target.
Comparison with Similar Compounds
Comparison with Structural Analogs
Structural Modifications and Substituent Effects
Key derivatives of tert-butyl 3,4-dihydroquinoxaline-1(2H)-carboxylate include substitutions at positions 6, 7, and 3, as well as modifications to the dihydroquinoxaline ring. Below is a comparative analysis of notable analogs:
Table 1: Structural and Physical Properties of Selected Derivatives
Key Observations :
- Electron-Withdrawing Groups (EWGs): The 6-nitro derivative (S-5) exhibits higher reactivity due to the NO₂ group, facilitating nucleophilic aromatic substitution (SNAr) reactions .
- Electron-Donating Groups (EDGs) : The 6-methoxy analog shows enhanced solubility in polar solvents, attributed to the OCH₃ group’s polarity .
Insights :
Biological Activity
Introduction
tert-Butyl 3,4-dihydroquinoxaline-1(2H)-carboxylate is a compound that has garnered attention in medicinal chemistry due to its potential biological activities. This article explores its synthesis, biological properties, and therapeutic applications based on recent research findings.
- Molecular Formula : C₁₃H₁₈N₂O₂
- Molecular Weight : 234.294 g/mol
- Density : 1.1 ± 0.1 g/cm³
- Boiling Point : 345.3 ± 22.0 °C at 760 mmHg
- Flash Point : 162.6 ± 22.3 °C
Synthesis
The synthesis of this compound typically involves the cyclization of appropriate precursors under specific conditions, often yielding high purity and yield rates. For instance, one synthetic route involves the reaction of substituted phenyl derivatives with dihydroquinoxaline intermediates, followed by carboxylation to obtain the target compound .
Antimicrobial Activity
Research indicates that quinoxaline derivatives, including this compound, exhibit significant antimicrobial properties. In particular:
- Antibacterial Activity : Several studies have shown that quinoxaline derivatives can inhibit the growth of various bacterial strains, including resistant strains. The mechanism often involves interference with bacterial DNA synthesis or cell wall formation .
- Antiviral Activity : The compound has demonstrated activity against viruses such as the Tobacco Mosaic Virus (TMV) with effective concentrations (EC50) significantly lower than those of standard antiviral agents .
Anticancer Potential
Quinoxaline derivatives have been evaluated for their anticancer properties:
- Cell Line Studies : In vitro studies have reported IC50 values in low micromolar ranges against various cancer cell lines. For instance, compounds derived from quinoxaline structures have shown IC50 values as low as 1.9 µg/mL against HCT-116 cells and 2.3 µg/mL against MCF-7 cells, indicating potent anticancer activity compared to conventional drugs like doxorubicin (IC50 = 3.23 µg/mL) .
The biological activities of this compound can be attributed to its ability to interact with specific biological targets:
- Enzyme Inhibition : Some studies suggest that quinoxaline derivatives act as inhibitors of key enzymes involved in cancer progression and microbial resistance mechanisms .
- Cellular Pathways : The compound may modulate signaling pathways related to apoptosis and cell proliferation, contributing to its anticancer effects .
Case Study 1: Antiviral Efficacy
In a study assessing the antiviral efficacy of various quinoxaline derivatives, this compound was found to reduce viral replication in infected cell cultures significantly. The EC50 was determined to be approximately 3.1 nM, showcasing its potential as a therapeutic agent against viral infections .
Case Study 2: Anticancer Activity
A comparative study involving different quinoxaline derivatives showed that this compound exhibited superior cytotoxicity against breast and colon cancer cell lines. The study highlighted the compound's ability to induce apoptosis through upregulation of pro-apoptotic factors while downregulating anti-apoptotic proteins .
Q & A
Basic Research Questions
Q. What are the common synthetic routes for tert-Butyl 3,4-dihydroquinoxaline-1(2H)-carboxylate, and what key reagents are involved?
The compound is synthesized via alkylation reactions. A representative method involves reacting this compound with propargyl bromide in the presence of potassium carbonate and sodium iodide in DMF at 50°C for 4 hours . Alternative routes may use Boc-protected intermediates and transition metal catalysts (e.g., Pd₂(dba)₃, BINAP) for functionalization . Key reagents include alkyl halides, bases (e.g., K₂CO₃), and polar aprotic solvents like DMF.
Q. How is this compound typically purified after synthesis?
Post-synthesis purification often employs preparative HPLC or column chromatography. For example, crude products are dissolved in methanol or acetonitrile and purified via reverse-phase HPLC with gradients of water and organic solvents. Purity is confirmed by LC-MS (e.g., [M−H]+ ion analysis) and NMR spectroscopy .
Q. What analytical methods are used to confirm the structural integrity of this compound?
- NMR spectroscopy : ¹H and ¹³C NMR are critical for confirming proton environments and carbon frameworks. For instance, characteristic peaks include tert-butyl protons at δ ~1.57 ppm and aromatic protons in the quinoxaline ring (δ 7.0–7.7 ppm) .
- LC-MS : High-resolution mass spectrometry (HRMS) validates molecular weight (e.g., [M−H]+ at m/z 396.1565) .
- X-ray crystallography : While not commonly reported for this compound, SHELX programs are widely used for small-molecule crystallography .
Advanced Research Questions
Q. How can researchers optimize the alkylation step to improve yields of derivatives?
- Reagent stoichiometry : Use excess alkylating agents (e.g., 2.0 eq propargyl bromide) to drive the reaction to completion .
- Catalysis : Add sodium iodide as a nucleophilic catalyst to enhance reactivity .
- Temperature control : Heating to 50°C accelerates the reaction while minimizing side products.
- Solvent selection : Polar solvents like DMF stabilize transition states and improve solubility .
Q. What strategies resolve contradictions between experimental NMR data and computational predictions?
- Cross-validation : Compare experimental data with density functional theory (DFT)-calculated chemical shifts.
- Decoupling experiments : Use 2D NMR (e.g., COSY, HSQC) to resolve overlapping signals .
- Isotopic labeling : Introduce deuterated analogs to simplify spectral interpretation.
- Crystallographic confirmation : If feasible, resolve ambiguities via single-crystal X-ray analysis .
Q. How can this compound be functionalized for bioactive analogue design?
- Amide coupling : React with hydroxamic acids or carboxylic acids to create enzyme inhibitors (e.g., histone deacetylase inhibitors) .
- Click chemistry : Introduce alkyne or azide groups for Huisgen cycloaddition to generate triazole-linked conjugates .
- Reductive amination : Modify the quinoxaline ring with amines for µ-opioid receptor agonist development .
Q. What are the challenges in scaling up the synthesis of this compound for in vivo studies?
Properties
IUPAC Name |
tert-butyl 3,4-dihydro-2H-quinoxaline-1-carboxylate | |
---|---|---|
Source | PubChem | |
URL | https://pubchem.ncbi.nlm.nih.gov | |
Description | Data deposited in or computed by PubChem | |
InChI |
InChI=1S/C13H18N2O2/c1-13(2,3)17-12(16)15-9-8-14-10-6-4-5-7-11(10)15/h4-7,14H,8-9H2,1-3H3 | |
Source | PubChem | |
URL | https://pubchem.ncbi.nlm.nih.gov | |
Description | Data deposited in or computed by PubChem | |
InChI Key |
DXXFPGTUHSMYSG-UHFFFAOYSA-N | |
Source | PubChem | |
URL | https://pubchem.ncbi.nlm.nih.gov | |
Description | Data deposited in or computed by PubChem | |
Canonical SMILES |
CC(C)(C)OC(=O)N1CCNC2=CC=CC=C21 | |
Source | PubChem | |
URL | https://pubchem.ncbi.nlm.nih.gov | |
Description | Data deposited in or computed by PubChem | |
Molecular Formula |
C13H18N2O2 | |
Source | PubChem | |
URL | https://pubchem.ncbi.nlm.nih.gov | |
Description | Data deposited in or computed by PubChem | |
DSSTOX Substance ID |
DTXSID70587575 | |
Record name | tert-Butyl 3,4-dihydroquinoxaline-1(2H)-carboxylate | |
Source | EPA DSSTox | |
URL | https://comptox.epa.gov/dashboard/DTXSID70587575 | |
Description | DSSTox provides a high quality public chemistry resource for supporting improved predictive toxicology. | |
Molecular Weight |
234.29 g/mol | |
Source | PubChem | |
URL | https://pubchem.ncbi.nlm.nih.gov | |
Description | Data deposited in or computed by PubChem | |
CAS No. |
887590-25-2 | |
Record name | tert-Butyl 3,4-dihydroquinoxaline-1(2H)-carboxylate | |
Source | EPA DSSTox | |
URL | https://comptox.epa.gov/dashboard/DTXSID70587575 | |
Description | DSSTox provides a high quality public chemistry resource for supporting improved predictive toxicology. | |
Record name | tert-butyl 1,2,3,4-tetrahydroquinoxaline-1-carboxylate | |
Source | European Chemicals Agency (ECHA) | |
URL | https://echa.europa.eu/information-on-chemicals | |
Description | The European Chemicals Agency (ECHA) is an agency of the European Union which is the driving force among regulatory authorities in implementing the EU's groundbreaking chemicals legislation for the benefit of human health and the environment as well as for innovation and competitiveness. | |
Explanation | Use of the information, documents and data from the ECHA website is subject to the terms and conditions of this Legal Notice, and subject to other binding limitations provided for under applicable law, the information, documents and data made available on the ECHA website may be reproduced, distributed and/or used, totally or in part, for non-commercial purposes provided that ECHA is acknowledged as the source: "Source: European Chemicals Agency, http://echa.europa.eu/". Such acknowledgement must be included in each copy of the material. ECHA permits and encourages organisations and individuals to create links to the ECHA website under the following cumulative conditions: Links can only be made to webpages that provide a link to the Legal Notice page. | |
Disclaimer and Information on In-Vitro Research Products
Please be aware that all articles and product information presented on BenchChem are intended solely for informational purposes. The products available for purchase on BenchChem are specifically designed for in-vitro studies, which are conducted outside of living organisms. In-vitro studies, derived from the Latin term "in glass," involve experiments performed in controlled laboratory settings using cells or tissues. It is important to note that these products are not categorized as medicines or drugs, and they have not received approval from the FDA for the prevention, treatment, or cure of any medical condition, ailment, or disease. We must emphasize that any form of bodily introduction of these products into humans or animals is strictly prohibited by law. It is essential to adhere to these guidelines to ensure compliance with legal and ethical standards in research and experimentation.