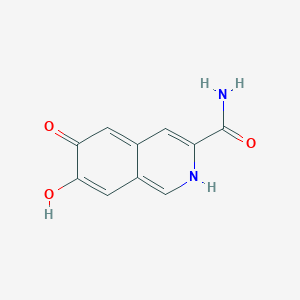
6,7-Dihydroxyisoquinoline-3-carboxamide
- Click on QUICK INQUIRY to receive a quote from our team of experts.
- With the quality product at a COMPETITIVE price, you can focus more on your research.
Overview
Description
7-Hydroxy-6-oxo-2H-isoquinoline-3-carboxamide is a heterocyclic compound with the molecular formula C10H8N2O3. It is a derivative of isoquinoline, a class of compounds known for their diverse biological activities and applications in medicinal chemistry.
Preparation Methods
The synthesis of 6,7-Dihydroxyisoquinoline-3-carboxamide typically involves the cyclization of appropriate precursors under specific conditions. One common method involves the reaction of anthranilic acid derivatives with suitable reagents to form the isoquinoline core, followed by functionalization to introduce the hydroxy and carboxamide groups . Industrial production methods may involve optimized reaction conditions, such as the use of catalysts and controlled temperatures, to achieve high yields and purity.
Chemical Reactions Analysis
7-Hydroxy-6-oxo-2H-isoquinoline-3-carboxamide undergoes various chemical reactions, including:
Oxidation: The hydroxy group can be oxidized to form quinone derivatives.
Reduction: The carbonyl group can be reduced to form alcohol derivatives.
Substitution: The compound can undergo nucleophilic substitution reactions, particularly at the carboxamide group. Common reagents used in these reactions include oxidizing agents like potassium permanganate, reducing agents like sodium borohydride, and nucleophiles like amines
Scientific Research Applications
Medicinal Chemistry
6,7-Dihydroxyisoquinoline-3-carboxamide has been investigated for its potential therapeutic properties, particularly in the development of new drugs. Its structure allows it to interact with various biological targets, making it a candidate for:
- Antimicrobial Agents : Research indicates that this compound may inhibit the growth of certain bacteria and fungi, suggesting its use as an antimicrobial agent.
- Anticancer Properties : Studies have shown that it can affect pathways involved in cancer cell proliferation and survival, indicating potential as an anticancer drug.
The compound exhibits various biological activities that are being explored:
- Enzyme Inhibition : It may inhibit enzymes involved in DNA replication and protein synthesis, which is crucial for its antimicrobial and anticancer effects.
- Receptor Interaction : The compound's ability to bind to specific receptors has been studied, which could lead to the development of targeted therapies.
Industrial Applications
In addition to its medicinal properties, this compound is utilized in industrial chemistry:
- Synthesis of Dyes and Pigments : Its chemical structure allows for modifications that lead to the formation of dyes and pigments used in various industries.
- Building Block in Organic Synthesis : It serves as a precursor for synthesizing more complex heterocyclic compounds, which are valuable in pharmaceuticals and agrochemicals.
Case Study 1: Anticancer Activity
A study explored the effects of this compound on cancer cell lines. The results showed that the compound significantly reduced cell viability in several types of cancer cells by inducing apoptosis through the inhibition of specific signaling pathways. This highlights its potential as a lead compound for developing new anticancer agents.
Case Study 2: Antimicrobial Properties
Research conducted on the antimicrobial effects of this compound demonstrated that it effectively inhibited the growth of both Gram-positive and Gram-negative bacteria. The mechanism was attributed to its ability to disrupt bacterial cell wall synthesis and function.
Mechanism of Action
The mechanism of action of 6,7-Dihydroxyisoquinoline-3-carboxamide involves its interaction with specific molecular targets and pathways. The compound can inhibit certain enzymes or receptors, leading to its biological effects. For example, it may inhibit the activity of enzymes involved in DNA replication or protein synthesis, thereby exerting antimicrobial or anticancer effects .
Comparison with Similar Compounds
7-Hydroxy-6-oxo-2H-isoquinoline-3-carboxamide can be compared with other similar compounds, such as:
4-Hydroxy-2-quinolones: These compounds also possess a hydroxy group and a quinoline core, but differ in their substitution patterns and biological activities.
Coumarin derivatives: These compounds have a similar heterocyclic structure and are known for their diverse biological activities.
Quinoline derivatives: These compounds share the quinoline core and are widely studied for their medicinal properties.
The uniqueness of 6,7-Dihydroxyisoquinoline-3-carboxamide lies in its specific substitution pattern, which imparts distinct chemical and biological properties.
Properties
CAS No. |
146515-42-6 |
---|---|
Molecular Formula |
C10H8N2O3 |
Molecular Weight |
204.18 g/mol |
IUPAC Name |
6,7-dihydroxyisoquinoline-3-carboxamide |
InChI |
InChI=1S/C10H8N2O3/c11-10(15)7-1-5-2-8(13)9(14)3-6(5)4-12-7/h1-4,13-14H,(H2,11,15) |
InChI Key |
OJUNRVBMXVTBNF-UHFFFAOYSA-N |
SMILES |
C1=C2C=C(N=CC2=CC(=C1O)O)C(=O)N |
Isomeric SMILES |
C1=C2C=C(NC=C2C=C(C1=O)O)C(=O)N |
Canonical SMILES |
C1=C2C=C(NC=C2C=C(C1=O)O)C(=O)N |
Synonyms |
3-Isoquinolinecarboxamide, 6,7-dihydroxy- (9CI) |
Origin of Product |
United States |
Disclaimer and Information on In-Vitro Research Products
Please be aware that all articles and product information presented on BenchChem are intended solely for informational purposes. The products available for purchase on BenchChem are specifically designed for in-vitro studies, which are conducted outside of living organisms. In-vitro studies, derived from the Latin term "in glass," involve experiments performed in controlled laboratory settings using cells or tissues. It is important to note that these products are not categorized as medicines or drugs, and they have not received approval from the FDA for the prevention, treatment, or cure of any medical condition, ailment, or disease. We must emphasize that any form of bodily introduction of these products into humans or animals is strictly prohibited by law. It is essential to adhere to these guidelines to ensure compliance with legal and ethical standards in research and experimentation.