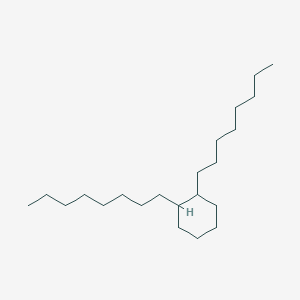
1,2-Dioctylcyclohexane
- Click on QUICK INQUIRY to receive a quote from our team of experts.
- With the quality product at a COMPETITIVE price, you can focus more on your research.
Overview
Description
1,2-Dioctylcyclohexane is an organic compound with the molecular formula C22H44 It is a derivative of cyclohexane, where two octyl groups are attached to the first and second carbon atoms of the cyclohexane ring
Preparation Methods
Synthetic Routes and Reaction Conditions: 1,2-Dioctylcyclohexane can be synthesized through several methods. One common approach involves the catalytic hydrogenation of dioctylcyclohexadiene. This reaction typically requires a hydrogenation catalyst such as palladium on carbon (Pd/C) and is carried out under high pressure and temperature conditions to ensure complete hydrogenation.
Industrial Production Methods: In industrial settings, the production of this compound often involves the use of continuous flow reactors to maintain consistent reaction conditions and high yields. The process may also include purification steps such as distillation and recrystallization to obtain the desired product with high purity.
Chemical Reactions Analysis
Types of Reactions: 1,2-Dioctylcyclohexane can undergo various chemical reactions, including:
Oxidation: This compound can be oxidized using strong oxidizing agents like potassium permanganate (KMnO4) or chromium trioxide (CrO3) to form corresponding ketones or carboxylic acids.
Reduction: Reduction reactions can be performed using reducing agents such as lithium aluminum hydride (LiAlH4) to convert any functional groups present into their corresponding alcohols.
Substitution: Halogenation reactions can introduce halogen atoms into the cyclohexane ring, using reagents like bromine (Br2) or chlorine (Cl2) under UV light or in the presence of a catalyst.
Common Reagents and Conditions:
Oxidation: KMnO4, CrO3, acidic or basic conditions.
Reduction: LiAlH4, sodium borohydride (NaBH4), in anhydrous solvents.
Substitution: Br2, Cl2, UV light, or catalysts like iron (Fe).
Major Products:
Oxidation: Ketones, carboxylic acids.
Reduction: Alcohols.
Substitution: Halogenated cyclohexanes.
Scientific Research Applications
1,2-Dioctylcyclohexane has several applications in scientific research, including:
Chemistry: Used as a solvent or intermediate in organic synthesis.
Biology: Studied for its potential interactions with biological membranes and proteins.
Medicine: Investigated for its potential use in drug delivery systems due to its hydrophobic nature.
Industry: Utilized in the production of specialty chemicals and materials, such as lubricants and plasticizers.
Mechanism of Action
The mechanism of action of 1,2-dioctylcyclohexane largely depends on its interactions with other molecules. In biological systems, it may interact with lipid bilayers, altering membrane fluidity and permeability. Its hydrophobic nature allows it to embed within lipid membranes, potentially affecting the function of membrane-bound proteins and signaling pathways.
Comparison with Similar Compounds
1,2-Dimethylcyclohexane: A smaller analog with methyl groups instead of octyl groups.
1,2-Diethylcyclohexane: Similar structure but with ethyl groups.
1,2-Dipropylcyclohexane: Contains propyl groups.
Uniqueness: 1,2-Dioctylcyclohexane is unique due to its long octyl chains, which impart distinct physical and chemical properties compared to its smaller analogs. These long chains increase its hydrophobicity and potential for interactions with lipid membranes, making it particularly useful in applications requiring hydrophobic interactions.
Properties
Molecular Formula |
C22H44 |
---|---|
Molecular Weight |
308.6 g/mol |
IUPAC Name |
1,2-dioctylcyclohexane |
InChI |
InChI=1S/C22H44/c1-3-5-7-9-11-13-17-21-19-15-16-20-22(21)18-14-12-10-8-6-4-2/h21-22H,3-20H2,1-2H3 |
InChI Key |
NTOKGSQOUBXQAJ-UHFFFAOYSA-N |
Canonical SMILES |
CCCCCCCCC1CCCCC1CCCCCCCC |
Origin of Product |
United States |
Disclaimer and Information on In-Vitro Research Products
Please be aware that all articles and product information presented on BenchChem are intended solely for informational purposes. The products available for purchase on BenchChem are specifically designed for in-vitro studies, which are conducted outside of living organisms. In-vitro studies, derived from the Latin term "in glass," involve experiments performed in controlled laboratory settings using cells or tissues. It is important to note that these products are not categorized as medicines or drugs, and they have not received approval from the FDA for the prevention, treatment, or cure of any medical condition, ailment, or disease. We must emphasize that any form of bodily introduction of these products into humans or animals is strictly prohibited by law. It is essential to adhere to these guidelines to ensure compliance with legal and ethical standards in research and experimentation.