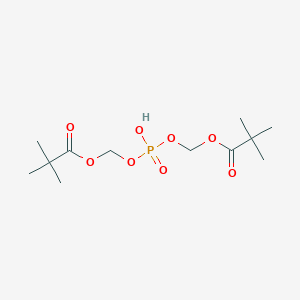
((Hydroxyphosphoryl)bis(oxy))bis(methylene) bis(2,2-dimethylpropanoate)
- Click on QUICK INQUIRY to receive a quote from our team of experts.
- With the quality product at a COMPETITIVE price, you can focus more on your research.
Overview
Description
((Hydroxyphosphoryl)bis(oxy))bis(methylene) bis(2,2-dimethylpropanoate): is a chemical compound with the molecular formula C12H23O8P and a molecular weight of 326.28 g/mol . This compound is known for its unique structure, which includes a phosphoryl group bonded to two oxygens, each connected to a methylene group, and further linked to two 2,2-dimethylpropanoate groups . It is primarily used in research settings and has various applications in chemistry and related fields .
Preparation Methods
Synthetic Routes and Reaction Conditions: The synthesis of ((Hydroxyphosphoryl)bis(oxy))bis(methylene) bis(2,2-dimethylpropanoate) involves the reaction of phosphoryl chloride with methylene glycol, followed by esterification with 2,2-dimethylpropanoic acid . The reaction typically requires an inert atmosphere and is conducted at low temperatures to prevent decomposition .
Industrial Production Methods: Industrial production of this compound follows similar synthetic routes but on a larger scale. The process involves stringent quality control measures, including purification steps such as recrystallization and chromatography to ensure high purity levels .
Chemical Reactions Analysis
Types of Reactions:
Reduction: Reduction reactions can target the phosphoryl group, converting it to a phosphine oxide.
Substitution: The ester groups can participate in nucleophilic substitution reactions, where the ester moiety is replaced by other nucleophiles.
Common Reagents and Conditions:
Oxidation: Common oxidizing agents include potassium permanganate and chromium trioxide.
Reduction: Reducing agents such as lithium aluminum hydride are used.
Substitution: Nucleophiles like amines and alcohols are commonly employed.
Major Products:
Oxidation: Carboxylic acids and phosphoric acids.
Reduction: Phosphine oxides and alcohols.
Substitution: Amides and ethers.
Scientific Research Applications
Chemistry:
- Used as a reagent in organic synthesis to introduce phosphoryl groups into molecules .
- Acts as a precursor for the synthesis of more complex organophosphorus compounds .
Biology:
Medicine:
- Explored for its potential use in drug delivery systems, particularly in targeting specific cellular pathways .
Industry:
Mechanism of Action
The mechanism of action of ((Hydroxyphosphoryl)bis(oxy))bis(methylene) bis(2,2-dimethylpropanoate) involves its interaction with molecular targets such as enzymes and receptors. The phosphoryl group can form strong hydrogen bonds and coordinate with metal ions, influencing various biochemical pathways . The ester groups can undergo hydrolysis, releasing active metabolites that further interact with cellular components .
Comparison with Similar Compounds
Phosphoric acid, bis(2,2-dimethylpropyl) ester: Similar structure but lacks the methylene groups.
Phosphinic acid, bis(2,2-dimethylpropyl) ester: Contains a phosphinic group instead of a phosphoryl group.
Phosphonic acid, bis(2,2-dimethylpropyl) ester: Features a phosphonic group, differing in oxidation state.
Uniqueness:
- The presence of both methylene and ester groups in ((Hydroxyphosphoryl)bis(oxy))bis(methylene) bis(2,2-dimethylpropanoate) provides unique reactivity and versatility in chemical reactions .
- Its ability to form stable complexes with metal ions makes it valuable in coordination chemistry and catalysis .
Properties
Molecular Formula |
C12H23O8P |
---|---|
Molecular Weight |
326.28 g/mol |
IUPAC Name |
[2,2-dimethylpropanoyloxymethoxy(hydroxy)phosphoryl]oxymethyl 2,2-dimethylpropanoate |
InChI |
InChI=1S/C12H23O8P/c1-11(2,3)9(13)17-7-19-21(15,16)20-8-18-10(14)12(4,5)6/h7-8H2,1-6H3,(H,15,16) |
InChI Key |
KBLSOCYEWZSBJM-UHFFFAOYSA-N |
Canonical SMILES |
CC(C)(C)C(=O)OCOP(=O)(O)OCOC(=O)C(C)(C)C |
Origin of Product |
United States |
Disclaimer and Information on In-Vitro Research Products
Please be aware that all articles and product information presented on BenchChem are intended solely for informational purposes. The products available for purchase on BenchChem are specifically designed for in-vitro studies, which are conducted outside of living organisms. In-vitro studies, derived from the Latin term "in glass," involve experiments performed in controlled laboratory settings using cells or tissues. It is important to note that these products are not categorized as medicines or drugs, and they have not received approval from the FDA for the prevention, treatment, or cure of any medical condition, ailment, or disease. We must emphasize that any form of bodily introduction of these products into humans or animals is strictly prohibited by law. It is essential to adhere to these guidelines to ensure compliance with legal and ethical standards in research and experimentation.