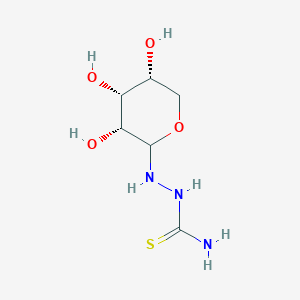
D-Ribopyranosyl thiosemicarbazide
- Click on QUICK INQUIRY to receive a quote from our team of experts.
- With the quality product at a COMPETITIVE price, you can focus more on your research.
Overview
Description
D-Ribopyranosyl thiosemicarbazide is a carbohydrate-derived thiosemicarbazide characterized by a β-D-ribopyranosyl moiety linked to a thiosemicarbazide functional group. Thiosemicarbazides are sulfur-containing analogs of semicarbazides, known for their diverse biological activities, including antimicrobial, antitumor, and enzyme inhibitory properties .
Preparation Methods
Synthetic Routes and Reaction Conditions
The synthesis of D-Ribopyranosyl thiosemicarbazide typically involves the reaction of D-ribose with thiosemicarbazide. The reaction is carried out under mild conditions, often in the presence of an acid catalyst to facilitate the formation of the thiosemicarbazone linkage . The reaction can be represented as follows:
D-ribose+thiosemicarbazide→D-Ribopyranosyl thiosemicarbazide
Industrial Production Methods
This includes optimizing reaction conditions, such as temperature, pH, and reaction time, to maximize yield and purity .
Chemical Reactions Analysis
Types of Reactions
D-Ribopyranosyl thiosemicarbazide undergoes various chemical reactions, including:
Oxidation: The compound can be oxidized to form corresponding sulfoxides or sulfones.
Reduction: Reduction reactions can convert the thiosemicarbazone group to a thiosemicarbazide group.
Common Reagents and Conditions
Oxidation: Common oxidizing agents include hydrogen peroxide and potassium permanganate.
Reduction: Reducing agents such as sodium borohydride or lithium aluminum hydride are used.
Substitution: Nucleophiles like amines or alcohols can be used in substitution reactions.
Major Products Formed
The major products formed from these reactions depend on the specific reagents and conditions used. For example, oxidation can yield sulfoxides or sulfones, while reduction can produce thiosemicarbazides .
Scientific Research Applications
D-Ribopyranosyl thiosemicarbazide has a wide range of scientific research applications:
Chemistry: It is used as a building block for synthesizing various heterocyclic compounds.
Biology: The compound exhibits antimicrobial and antifungal activities, making it useful in biological studies.
Medicine: Due to its anticancer properties, it is investigated for potential therapeutic applications in cancer treatment.
Industry: It is used in the development of new materials with specific properties, such as sensors and catalysts
Mechanism of Action
The mechanism of action of D-Ribopyranosyl thiosemicarbazide involves its interaction with specific molecular targets. For instance, it can inhibit the activity of certain enzymes, such as carbonic anhydrase, by binding to their active sites. This inhibition can disrupt various cellular processes, leading to the compound’s antimicrobial and anticancer effects .
Comparison with Similar Compounds
Comparison with Similar Thiosemicarbazide Derivatives
Thiosemicarbazide derivatives exhibit varied bioactivities depending on substituents, tautomeric forms, and stereochemistry. Below is a comparative analysis of D-ribopyranosyl thiosemicarbazide analogs and other structurally related compounds:
Structural and Tautomeric Variations
- Glycosylated Derivatives: N-(β-D-Galactopyranosyl)-thiosemicarbazide: Exhibits potent antibacterial activity against Staphylococcus epidermidis and Streptococcus mutans (MIC: 2–8 µg/mL) and low cytotoxicity (therapeutic index >10). N-(β-D-Xylopyranosyl)-thiosemicarbazide: Less active than its galactopyranosyl counterpart, with weaker antituberculosic (Pa = 0.65) and antineoplastic (Pa = 0.53) properties . Key Difference: The configuration of the sugar moiety (e.g., galactose vs. xylose) significantly impacts bioactivity. For example, acetylated glucose derivatives in tyrosinase inhibitors show IC₅₀ values as low as 0.31 µM, highlighting the role of stereochemistry .
- Aromatic and Heterocyclic Derivatives: Pyridinecarbonyl Thiosemicarbazides: Derivatives like 4-(2,4-dichlorophenyl)-1-(pyridin-3-yl)carbonyl thiosemicarbazide demonstrate antiproliferative activity against breast adenocarcinoma (IC₅₀: 12 µM) and hepatocellular carcinoma (IC₅₀: 15 µM) cell lines . 4-Nitrophenyl Thiosemicarbazides: Exhibit dual α-glucosidase inhibition (IC₅₀: 18–25 µM) and antibacterial activity against Bacillus subtilis (MIC: 4 µg/mL) .
Mechanistic and Computational Insights
- Tautomerism : Thiosemicarbazides predominantly exist in the 01 tautomeric form (carbonyl and thione groups) in crystalline states. Ionization shifts equilibrium toward the 02 form , affecting reactivity and target binding .
- Molecular Docking: Pyridinecarbonyl derivatives bind to α-glucosidase similarly to glucose, while galactopyranosyl analogs target bacterial D-alanine-D-alanine ligase, disrupting cell wall synthesis .
Biological Activity
D-Ribopyranosyl thiosemicarbazide is a compound that has garnered interest in medicinal chemistry due to its potential biological activities, particularly its antibacterial and antiviral properties. This article provides a comprehensive overview of its biological activity, supported by data tables, case studies, and detailed research findings.
Chemical Structure and Synthesis
This compound is derived from the thiosemicarbazide class of compounds, which are known for their diverse biological activities. The synthesis typically involves the reaction of ribose derivatives with thiosemicarbazide under acidic or basic conditions. The resulting compound features a ribose sugar moiety linked to a thiosemicarbazide functional group, which is crucial for its biological activity.
Antibacterial Activity
Research indicates that this compound exhibits significant antibacterial properties. A study conducted on various glycosylthiosemicarbazides, including D-Ribopyranosyl derivatives, showed promising results against several bacterial strains.
- In vitro Studies : In vitro experiments demonstrated that this compound has a pronounced bactericidal effect against pathogenic microorganisms, including Escherichia coli and Salmonella species. The minimum inhibitory concentration (MIC) values were determined through serial dilution methods, revealing effective concentrations that inhibit bacterial growth.
Table 1: Antibacterial Activity of this compound
Bacterial Strain | MIC (µg/mL) | Bactericidal/Bacteriostatic |
---|---|---|
Escherichia coli | 1:380 | Bacteriostatic |
Salmonella spp. | 1:640 | Bactericidal |
These findings suggest that this compound could be developed as a therapeutic agent for treating infections caused by resistant bacterial strains.
Antiviral Activity
This compound has also been evaluated for its antiviral properties. Preliminary studies indicate potential efficacy against viral pathogens, although further research is needed to elucidate the mechanisms involved.
- Case Study : In a controlled study, the compound was tested against influenza virus strains. Results suggested a reduction in viral replication, indicating that this compound may interfere with viral life cycles.
Toxicity Assessment
The safety profile of this compound was assessed through various toxicological studies. The compound was classified as belonging to the III class of hazardous substances based on its toxicity levels observed in animal models.
Table 2: Toxicity Profile of this compound
Test Subject | Observed Effects | Toxicity Class |
---|---|---|
Rodents | Mild gastrointestinal distress | Class III |
Aquatic Species | Minimal impact | Non-toxic |
These assessments are crucial for determining the viability of this compound as a therapeutic agent.
The mechanism by which this compound exerts its biological effects is believed to involve interference with cellular processes in bacteria and viruses. Thiosemicarbazides are known to inhibit key metabolic pathways, such as nucleic acid synthesis and enzyme activity, which are essential for microbial growth and replication.
Q & A
Q. What are the common synthetic routes for preparing D-Ribopyranosyl thiosemicarbazide derivatives?
Basic
Synthesis typically involves condensation reactions between pyridinecarbonyl hydrazides and substituted isothiocyanates. For example, derivatives can be synthesized by reacting 2-, 3-, or 4-pyridinecarboxylic acid hydrazides with aryl isothiocyanates (e.g., 4-methylphenyl or 4-nitrophenyl isothiocyanate) in ethanol or DMSO under reflux. Purification is achieved via recrystallization, and structural confirmation employs elemental analysis, NMR, and IR spectroscopy .
Q. Which spectroscopic and crystallographic methods are critical for characterizing thiosemicarbazide derivatives?
Basic
Key techniques include:
- NMR spectroscopy : To identify NH proton signals (9–11 ppm) and confirm tautomeric forms.
- IR spectroscopy : Bands at ~1700 cm⁻¹ (C=O) and ~1300 cm⁻¹ (C=S) validate the keto-thione tautomer.
- X-ray crystallography : Resolves bond lengths (e.g., C=O: 1.22 Å; C=S: 1.68 Å) and intermolecular interactions (e.g., N–H···S hydrogen bonds) .
Q. How does tautomerism influence the biological activity of this compound derivatives?
Advanced
Thiosemicarbazides exhibit keto-thione tautomerism, where the keto-thione form (with C=O and C=S groups) is most stable in polar solvents and solid states. This tautomer impacts binding to targets like enzymes or DNA by altering hydrogen-bonding capacity and electrostatic complementarity. Computational studies (DFT) and experimental data (NMR/X-ray) confirm that tautomer stability correlates with antibacterial activity (e.g., MIC values of 7.81–62.5 µg/mL against Streptococcus spp.) .
Q. What computational approaches predict the electronic properties of thiosemicarbazide derivatives?
Advanced
Density Functional Theory (DFT) at the B3LYP/6-311++G(3df,3pd) level calculates:
- HOMO-LUMO gaps : Lower gaps (~4–5 eV) indicate higher reactivity.
- Electrostatic potential maps : Identify nucleophilic/electrophilic regions (e.g., pyridyl nitrogen as electronegative sites).
- Non-covalent interaction (NCI) analysis : Visualizes π-π stacking and hydrogen bonds in crystal packing .
Q. How are molecular docking studies applied to evaluate thiosemicarbazide-protein interactions?
Advanced
Docking software (e.g., AutoDock Vina) models ligand binding to targets like human serum albumin (HSA) or carbonic anhydrase. Parameters include:
- Binding affinity : Correlated with experimental inhibition constants (e.g., IC₅₀ values).
- Hydrogen-bond networks : Predict interactions with catalytic residues (e.g., N–H···O bonds with HSA’s Trp214).
- Conformational stability : Molecular dynamics simulations validate docking poses .
Q. What methodologies assess the antibacterial efficacy of thiosemicarbazide derivatives?
Basic
- Minimum Inhibitory Concentration (MIC) assays : Test against Gram-positive (Staphylococcus aureus) and Gram-negative (E. coli) strains.
- Therapeutic Index (TI) : Compares cytotoxicity (e.g., IC₅₀ on Vero cells) vs. antimicrobial activity.
- Time-kill kinetics : Monitors bactericidal effects over 24 hours .
Q. How do substituent modifications on the thiosemicarbazide scaffold affect pharmacokinetics?
Advanced
- Lipophilicity : Electron-withdrawing groups (e.g., NO₂) enhance membrane penetration but reduce solubility.
- Steric effects : Bulky substituents (e.g., 2,4-dichlorophenyl) improve target specificity but may hinder metabolic clearance.
- SAR studies : Quantitative structure-activity relationship (QSAR) models link substituent Hammett constants (σ) to bioactivity .
Q. What role do non-covalent interactions play in stabilizing thiosemicarbazide crystal structures?
Advanced
X-ray data reveal:
Properties
Molecular Formula |
C6H13N3O4S |
---|---|
Molecular Weight |
223.25 g/mol |
IUPAC Name |
[[(3R,4R,5R)-3,4,5-trihydroxyoxan-2-yl]amino]thiourea |
InChI |
InChI=1S/C6H13N3O4S/c7-6(14)9-8-5-4(12)3(11)2(10)1-13-5/h2-5,8,10-12H,1H2,(H3,7,9,14)/t2-,3-,4-,5?/m1/s1 |
InChI Key |
IPZPYSHFPHKLJM-SOOFDHNKSA-N |
Isomeric SMILES |
C1[C@H]([C@H]([C@H](C(O1)NNC(=S)N)O)O)O |
Canonical SMILES |
C1C(C(C(C(O1)NNC(=S)N)O)O)O |
Origin of Product |
United States |
Disclaimer and Information on In-Vitro Research Products
Please be aware that all articles and product information presented on BenchChem are intended solely for informational purposes. The products available for purchase on BenchChem are specifically designed for in-vitro studies, which are conducted outside of living organisms. In-vitro studies, derived from the Latin term "in glass," involve experiments performed in controlled laboratory settings using cells or tissues. It is important to note that these products are not categorized as medicines or drugs, and they have not received approval from the FDA for the prevention, treatment, or cure of any medical condition, ailment, or disease. We must emphasize that any form of bodily introduction of these products into humans or animals is strictly prohibited by law. It is essential to adhere to these guidelines to ensure compliance with legal and ethical standards in research and experimentation.