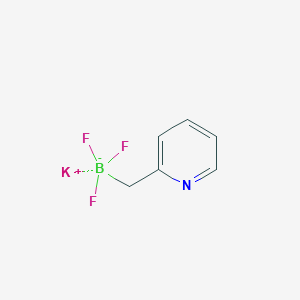
Potassium cyclobutyloxymethyltrifluoroborate
- Click on QUICK INQUIRY to receive a quote from our team of experts.
- With the quality product at a COMPETITIVE price, you can focus more on your research.
Overview
Description
Potassium cyclobutyloxymethyltrifluoroborate is a member of the organotrifluoroborate family, a class of compounds widely used in cross-coupling reactions due to their stability, solubility, and compatibility with transition-metal catalysts. This compound features a cyclobutyloxymethyl group attached to a trifluoroborate anion, balanced by a potassium counterion. Its synthesis typically involves an SN2 displacement reaction between potassium bromomethyltrifluoroborate and cyclobutanolate, followed by purification via continuous Soxhlet extraction to overcome low solubility in organic solvents .
The cyclobutyl group introduces unique steric and electronic properties, including ring strain, which can enhance reactivity in certain transformations compared to larger cycloalkyl analogs. This compound has been employed in palladium-catalyzed Suzuki-Miyaura cross-couplings to access functionalized aromatic and heteroaromatic systems, though its applications are less documented than those of simpler analogs like potassium methoxymethyltrifluoroborate .
Preparation Methods
Synthetic Routes and Reaction Conditions
The preparation of potassium cyclobutyloxymethyltrifluoroborate typically involves the reaction of cyclobutyloxymethylboronic acid with potassium bifluoride (KHF2). The reaction is carried out under controlled conditions to ensure the formation of the desired trifluoroborate salt. The general reaction scheme is as follows:
Cyclobutyloxymethylboronic acid+KHF2→Potassium cyclobutyloxymethyltrifluoroborate
Industrial Production Methods
Industrial production of this compound follows similar synthetic routes but on a larger scale. The process involves the use of high-purity reagents and controlled environments to ensure consistent product quality. The reaction is typically carried out in large reactors with precise temperature and pressure controls.
Chemical Reactions Analysis
Types of Reactions
Potassium cyclobutyloxymethyltrifluoroborate undergoes various types of chemical reactions, including:
Substitution Reactions: It can participate in nucleophilic substitution reactions where the trifluoroborate group is replaced by other nucleophiles.
Cross-Coupling Reactions: It is commonly used in Suzuki-Miyaura cross-coupling reactions to form carbon-carbon bonds.
Oxidation and Reduction: While less common, it can also undergo oxidation and reduction reactions under specific conditions.
Common Reagents and Conditions
Nucleophilic Substitution: Common reagents include alkyl halides and nucleophiles such as amines or thiols.
Suzuki-Miyaura Coupling: Palladium catalysts and bases like potassium carbonate are typically used.
Oxidation and Reduction: Reagents such as hydrogen peroxide or sodium borohydride can be employed.
Major Products
The major products formed from these reactions depend on the specific reagents and conditions used. For example, in Suzuki-Miyaura coupling, the product is typically a biaryl compound.
Scientific Research Applications
Cross-Coupling Reactions
Potassium cyclobutyloxymethyltrifluoroborate is primarily utilized in cross-coupling reactions, which are fundamental in constructing complex organic molecules. These reactions allow for the formation of carbon-carbon bonds, facilitating the synthesis of pharmaceuticals and agrochemicals.
- Mechanism : The compound acts as a nucleophile in palladium-catalyzed cross-coupling reactions. It can react with various electrophiles such as halides and acyl chlorides under mild conditions, often employing photoredox catalysis to enhance yield and selectivity .
- Case Study : A study demonstrated the successful use of this compound in coupling with methyl 4-bromobenzoate, achieving a yield of 92% under optimized conditions. This highlights its efficiency in forming desired products while minimizing by-products .
Medicinal Chemistry
In medicinal chemistry, the trifluoroborate moiety has been shown to enhance the pharmacological properties of drugs.
- Drug Development : The incorporation of the trifluoromethyl-cyclobutyl group into drug candidates has been linked to improved lipophilicity and metabolic stability. For instance, replacing a tert-butyl group with a trifluoromethyl-cyclobutane group resulted in significant increases in log D (a measure of lipophilicity) across several bioactive compounds .
- Example Compounds : In model compounds like Butenafine and Pivhydrazine, this substitution maintained water solubility while enhancing other pharmacokinetic parameters. These modifications are crucial for optimizing drug candidates for better therapeutic efficacy .
Materials Science
The unique properties of this compound make it suitable for applications in materials science.
- Polymer Chemistry : It can be utilized in the synthesis of functional polymers through cross-linking reactions. The trifluoroborate group provides stability and enhances the mechanical properties of polymeric materials .
- Nanomaterials : Research indicates that incorporating this compound into nanomaterials can improve their electrical conductivity and thermal stability, making them suitable for advanced electronic applications.
Environmental Chemistry
This compound also finds applications in environmental chemistry, particularly in the development of sustainable chemical processes.
- Green Chemistry : Its use in photoredox catalysis exemplifies green chemistry principles by allowing reactions to occur under ambient conditions with reduced waste generation . This aligns with current trends towards environmentally friendly synthetic methodologies.
Summary Table of Applications
Mechanism of Action
The mechanism by which potassium cyclobutyloxymethyltrifluoroborate exerts its effects is primarily through its reactivity as a nucleophile. In cross-coupling reactions, it acts as a nucleophilic partner, forming bonds with electrophilic species. The molecular targets and pathways involved depend on the specific reaction and application.
Comparison with Similar Compounds
Structural Analogs and Their Reactivity
The table below compares potassium cyclobutyloxymethyltrifluoroborate with structurally related organotrifluoroborates:
Key Observations :
- Steric Effects : The cyclobutyloxymethyl group imposes greater steric hindrance than cyclopentylmethyl or methoxymethyl groups, slowing transmetalation steps in cross-couplings .
- Ring Strain : Cyclopropane derivatives exhibit higher reactivity due to ring strain, whereas cyclobutane derivatives balance strain and steric bulk .
- Solubility : Bulky or polar substituents (e.g., hydroxyethylphenyl) reduce solubility, complicating purification and reaction setup .
Cross-Coupling Performance
This compound has been cross-coupled with aryl chlorides under palladium catalysis (e.g., Pd(OAc)₂, SPhos), yielding biaryl products in 40–75% efficiency, depending on the substrate . In contrast:
- Potassium alkenyltrifluoroborates achieve >80% yields in couplings with 2-chloroacetates under similar conditions .
- Potassium methoxymethyltrifluoroborate reacts efficiently even with electron-deficient aryl chlorides, achieving >90% yields due to minimal steric interference .
Functional Group Compatibility
The cyclobutyloxymethyl group is less prone to side reactions (e.g., oxidation) compared to azidomethyl or cyanomethyl analogs (e.g., potassium 2-azidomethylphenyltrifluoroborate), which require careful handling to avoid decomposition . However, it is more sensitive to strong acids/bases than alkyl-substituted trifluoroborates like potassium cyclohexyltrifluoroborate .
Biological Activity
Potassium cyclobutyloxymethyltrifluoroborate is a compound that has garnered interest in medicinal chemistry due to its unique structural properties and potential biological activities. This article reviews the biological activity of this compound, highlighting its physicochemical characteristics, metabolic stability, and effects on various biological systems.
The physicochemical properties of this compound are crucial for understanding its biological activity. The trifluoromethyl-cyclobutyl group exhibits increased steric size and lipophilicity compared to traditional groups like tert-butyl. This modification can enhance the compound's interaction with biological targets while maintaining or improving its metabolic stability.
Property | Value/Description |
---|---|
Steric Size | Increased due to trifluoromethyl group |
Lipophilicity (log D) | Increased by 0.4-0.5 units |
Water Solubility | Slightly decreased |
Metabolic Stability | Variable effects depending on analogues |
Antifungal Activity
Research indicates that this compound and its analogues exhibit antifungal properties. For instance, studies on the antifungal agent butenafine, which contains a similar trifluoromethyl-cyclobutane structure, demonstrated effective inhibition against Trichophyton mentagrophytes and Trichophyton rubrum. Although the original drug was slightly more potent, the CF3-cyclobutane analogues maintained significant antifungal activity, suggesting that structural modifications can lead to viable therapeutic alternatives without sacrificing efficacy .
Metabolic Stability Studies
The metabolic stability of this compound was assessed through various model compounds. The incorporation of the trifluoromethyl-cyclobutane moiety often led to inconsistent metabolic stability outcomes. For example, in certain compounds, this modification resulted in decreased metabolic clearance rates, while in others, it enhanced stability . This variability underscores the need for detailed studies on specific analogues to predict their behavior in biological systems accurately.
Case Studies
- Butenafine Analogues : The antifungal activity of butenafine and its CF3-cyclobutane analogue was evaluated using disk diffusion methods against fungal strains. The results indicated that while the original compound was more effective, the analogue still exhibited significant antifungal properties.
- Buclizine Analogues : Similar studies were conducted with buclizine and its CF3-cyclobutane analogue. The findings suggested that structural modifications could retain antihistaminic activity while potentially reducing side effects associated with traditional antihistamines .
Q & A
Basic Research Questions
Q. What are the optimal synthetic conditions for preparing potassium cyclobutyloxymethyltrifluoroborate?
Methodological Answer: The compound is synthesized via SN2 displacement of potassium bromomethyltrifluoroborate with cyclobutyl alkoxides. Key steps include:
- Reagent Ratios : Use 3 equivalents of the alkoxide for efficient substitution .
- Isolation Challenges : Due to low solubility in organic solvents (e.g., acetone), continuous Soxhlet extraction is employed to separate the product from inorganic byproducts, improving yields to >90% .
- Scalability : Batches up to 100 g can be prepared using optimized protocols, ensuring high purity for downstream applications .
Q. What analytical techniques are recommended for characterizing this compound?
Methodological Answer: Standard characterization involves:
- Nuclear Magnetic Resonance (NMR) : 1H, 13C, 11B, and 19F NMR to confirm structure and boron coordination .
- High-Resolution Mass Spectrometry (HRMS) : Validates molecular weight and purity .
- X-ray Diffraction (XRD) : Resolves crystal structure and bonding geometry in solid-state studies .
Advanced Research Questions
Q. How can cross-coupling reactions involving this compound be optimized for complex molecule synthesis?
Methodological Answer: this compound is valuable in Suzuki-Miyaura cross-coupling . Optimization strategies include:
- Catalyst Selection : Palladium catalysts (e.g., Pd(OAc)2 with SPhos ligand) enhance coupling efficiency with aryl chlorides .
- Reaction Conditions : Use polar aprotic solvents (e.g., THF) at 80–100°C. Additives like Cs2CO3 improve transmetallation kinetics .
- Substrate Scope : Test steric and electronic effects of aryl partners; electron-deficient aryl chlorides typically yield higher conversions .
Q. How should researchers address contradictions in reported reaction yields or scalability?
Methodological Answer: Discrepancies often arise from:
- Solubility Variability : Differences in alkoxide solubility affect substitution efficiency. Pre-solubilizing alkoxides in DMF or DMSO can mitigate this .
- Byproduct Management : Incomplete removal of KBr during isolation reduces purity. Validate via ion chromatography or conductivity measurements .
- Scale-Up Artifacts : Larger batches may require adjusted heating rates or stirring dynamics. Pilot studies at 10–50 g scales are recommended before 100 g syntheses .
Q. What computational tools predict the reactivity of this compound in new reactions?
Methodological Answer:
- Retrosynthetic Analysis : Tools like Pistachio and Reaxys databases model feasible reaction pathways, prioritizing steps with high thermodynamic favorability .
- Density Functional Theory (DFT) : Calculates transition-state energies for SN2 displacement or cross-coupling steps, guiding catalyst/ligand selection .
- Machine Learning (ML) : Platforms trained on organoboron reaction datasets predict optimal solvents, temperatures, and catalysts for novel transformations .
Q. Safety and Handling
Q. What safety protocols are critical for handling this compound?
Methodological Answer:
- Storage : Keep at 2–8°C in airtight containers to prevent hydrolysis. Desiccate to avoid moisture absorption .
- Waste Disposal : Neutralize with aqueous bicarbonate before disposal at certified facilities. Avoid incineration due to HF release risks .
- Personal Protective Equipment (PPE) : Use nitrile gloves, goggles, and lab coats. Conduct reactions in a fume hood to manage volatile byproducts .
Properties
Molecular Formula |
C6H6BF3KN |
---|---|
Molecular Weight |
199.03 g/mol |
IUPAC Name |
potassium;trifluoro(pyridin-2-ylmethyl)boranuide |
InChI |
InChI=1S/C6H6BF3N.K/c8-7(9,10)5-6-3-1-2-4-11-6;/h1-4H,5H2;/q-1;+1 |
InChI Key |
OVXAIPOHXPUSOK-UHFFFAOYSA-N |
Canonical SMILES |
[B-](CC1=CC=CC=N1)(F)(F)F.[K+] |
Origin of Product |
United States |
Disclaimer and Information on In-Vitro Research Products
Please be aware that all articles and product information presented on BenchChem are intended solely for informational purposes. The products available for purchase on BenchChem are specifically designed for in-vitro studies, which are conducted outside of living organisms. In-vitro studies, derived from the Latin term "in glass," involve experiments performed in controlled laboratory settings using cells or tissues. It is important to note that these products are not categorized as medicines or drugs, and they have not received approval from the FDA for the prevention, treatment, or cure of any medical condition, ailment, or disease. We must emphasize that any form of bodily introduction of these products into humans or animals is strictly prohibited by law. It is essential to adhere to these guidelines to ensure compliance with legal and ethical standards in research and experimentation.