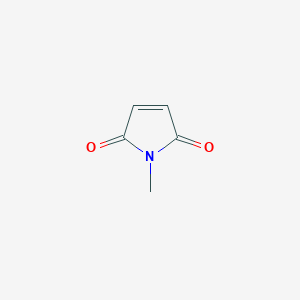
N-Methylmaleimide
Overview
Description
N-Methylmaleimide (NMM) is a maleimide derivative characterized by a methyl group attached to the nitrogen atom of the maleimide ring. This compound is widely utilized in organic synthesis, polymer chemistry, and biochemical studies due to its electrophilic α,β-unsaturated carbonyl structure, which enables reactions such as Michael additions, Diels-Alder cycloadditions, and thiol alkylation . Its applications range from synthesizing functionalized polymers to serving as a substrate in enzyme kinetics and a thiol-blocking agent in biochemical assays .
Preparation Methods
Cyclodehydration of N-Methylmaleamic Acid
The cyclodehydration of N-methylmaleamic acid represents a foundational approach for synthesizing N-methylmaleimide. This method involves the thermal elimination of water from the maleamic acid precursor, typically facilitated by acidic or catalytic conditions.
Traditional Acetic Anhydride-Mediated Dehydration
Early methods utilized acetic anhydride and alkali metal acetates (e.g., sodium or potassium acetate) at elevated temperatures (~80°C). However, these conditions proved suboptimal for aliphatic substituents like methyl groups, often yielding resinous by-products and low recoveries (50–60%) . For instance, the reaction of N-methylmaleamic acid with acetic anhydride and sodium acetate at 80°C for 3 hours produced this compound in only 58% yield, alongside 15–20% polymeric residues .
Tertiary Amine-Enhanced Cyclodehydration
A patent by introduced a modified protocol using tertiary amines (e.g., triethylamine) and lower fatty acid anhydrides (e.g., acetic anhydride) to suppress by-product formation. The mechanism involves the tertiary amine neutralizing liberated carboxylic acid groups, driving the equilibrium toward imide formation.
Reaction Conditions :
-
Molar ratio : 2–10 moles of triethylamine per mole of N-methylmaleamic acid
-
Temperature : 25–100°C
-
Solvent : Dichloromethane or tetrahydrofuran (THF)
Under these conditions, yields improved to 75–80% with >90% purity. For example, reacting 1 mole of N-methylmaleamic acid with 5 moles of triethylamine and 2 moles of acetic anhydride in THF at 50°C for 2 hours afforded this compound in 78% yield .
Catalytic Dehydration Using Acid Catalysts
Strong acid catalysts, such as orthophosphoric acid (H₃PO₄), have been instrumental in streamlining this compound synthesis. A patent by details a two-step process involving maleic anhydride and methylamine gas, followed by acid-catalyzed cyclization.
Reaction Protocol and Optimization
-
Maleamic Acid Formation : Maleic anhydride reacts with methylamine gas in chlorobenzene at 30°C to form N-methylmaleamic acid.
-
Cyclization : The slurry is treated with orthophosphoric acid (22.5 g), phenothiazine (0.1 g, radical inhibitor), and zinc acetate (0.02 g, co-catalyst) at 134°C for 2 hours.
Key Data :
-
Yield : 72.5% (first cycle), increasing to 86.4% upon catalyst reuse
-
Purity : 92.1–93.4% (HPLC analysis)
-
By-products : <5% oligomeric species
Catalyst Recycling and Efficiency
Recycling the separated orthophosphoric acid layer reduced raw material consumption by 40%. After three cycles, catalytic activity remained stable, with no detectable leaching .
Table 1: Impact of Catalyst Recycling on Yield
Cycle | Yield (%) | Purity (%) |
---|---|---|
1 | 72.5 | 92.1 |
2 | 86.4 | 93.4 |
3 | 85.9 | 93.2 |
Solvent and Reaction Condition Optimization
Solvent choice critically influences reaction kinetics and product isolation. Polar aprotic solvents (e.g., acetonitrile) enhance maleamic acid solubility, while high-boiling solvents (e.g., o-xylene) facilitate azeotropic water removal.
Chlorobenzene vs. Xylene
-
Chlorobenzene : Enabled 86.4% yield at 134°C due to efficient water separation .
-
Xylene : Lower yields (72–75%) attributed to incomplete maleamic acid dissolution .
Temperature and Time Dependence
Optimal cyclization occurs at 130–150°C. Prolonged heating (>3 hours) at >150°C degraded the product, reducing yields by 10–15% .
Analysis of By-products and Purity
Resinous by-products, a historical challenge in maleimide synthesis, are minimized through:
-
Radical Inhibitors : Phenothiazine (0.1 wt%) reduced oligomerization from 15% to <3% .
-
Acid Catalysts : Orthophosphoric acid suppressed side reactions better than HCl or H₂SO₄ .
Purity Enhancement Techniques :
-
Vacuum Distillation : Isolated this compound with 99.8% purity after removing chlorobenzene at 5 mmHg .
-
Crystallization : Recrystallization from ethyl acetate increased purity from 92.1% to 98.5% .
Comparative Evaluation of Methods
Table 2: Method Comparison for this compound Synthesis
Chemical Reactions Analysis
Diels-Alder and Retro-Diels-Alder (rDA) Reactions
NMM acts as a dienophile in Diels-Alder (DA) reactions, forming thermally reversible adducts with dienes like furan derivatives.
Key Findings:
-
Activation Parameters :
Parameter NMM-Furan Adduct Maleic Anhydride-Furan Adduct Activation Energy () 118 kJ/mol 103 kJ/mol Δ‡H° 115 kJ/mol 100 kJ/mol Δ‡S° -45 J/(mol·K) -55 J/(mol·K) The higher and Δ‡H° for NMM adducts indicate greater thermal stability compared to maleic anhydride analogs, attributed to reduced electron-withdrawing capacity of the N-methylimide group .
-
Solvent Effects :
NMM adducts show lower solvent polarity dependence than maleic anhydride derivatives. In tetrachloroethane (TCE), Δ‡H° for NMM adducts varies by <5 kJ/mol across solvents, versus >15 kJ/mol for maleic anhydride . -
Endo/Exo Isomerism :
DA reactions with furans produce kinetic (endo) and thermodynamic (exo) adducts. Exo isomers dominate under prolonged heating (70°C for 72 hours), with retro-DA temperatures () of 120°C (exo) vs. 100°C (endo) .
Thiol Addition Mechanisms
NMM undergoes thiol-Michael additions with alkyl/aryl thiols, critical for bioconjugation and polymer networks.
Experimental Insights :
-
Reaction Pathways :
-
Alkyl Thiols : Follow a stepwise mechanism with rate-limiting nucleophilic attack (, Δ‡S = -80 J/(mol·K)).
-
Aryl Thiols : Proceed via a concerted pathway with partial proton transfer, evidenced by solvent kinetic isotope effects (SKIE = 1.8).
-
-
Kinetic Parameters :
Thiol Type (M⁻¹s⁻¹, 25°C) Δ‡H (kJ/mol) Δ‡S (J/(mol·K)) Alkyl 0.12 65 -80 Aryl 0.04 75 -60 Ionic strength effects confirm charge development in the transition state for aryl thiols ( M⁻¹ slope in Debye-Hückel plots) .
Case Study: Thermally Reversible Polyurethanes
-
Synthesis :
NMM reacts with furan-functionalized cyclocarbonates (FCE) to form DA adducts. Optimal conditions:-
Temperature : 50°C
-
Time : 72 hours
-
Exo/Endo Ratio : 4:1 (achieved via precipitation in diethyl ether).
-
-
Thermal Reversibility :
Heating to 100°C in DMF triggers rDA, cleaving polymers into fragments (Fig. 1). SEC analysis shows:Time (h) (g/mol) Dispersity (Đ) 0 15,000 1.2 2 5,000 1.5 -
NMR Monitoring :
Comparative Reactivity in Click Chemistry
Scientific Research Applications
Thiol Blocking Reagent
N-Methylmaleimide is widely used in protein chemistry as a thiol-blocking agent. Its ability to react with free thiol groups makes it valuable for:
- Studying Protein Structure : By blocking thiol groups, researchers can investigate the role of specific cysteine residues in protein folding and stability.
- Enzyme Inhibition Studies : NMM is utilized to selectively inhibit enzymes that contain cysteine residues in their active sites, allowing for detailed kinetic studies.
Case Study : A study demonstrated that NMM effectively inhibited the enzyme activity of certain thiol-dependent enzymes, providing insights into their mechanisms of action .
Drug Delivery Systems
This compound has been integrated into drug delivery systems, particularly in the development of polymeric carriers that enhance solubility and stability of therapeutic agents. Its incorporation into cyclodextrin-based systems has shown promising results in improving the bioavailability of poorly soluble drugs.
- Enhanced Drug Solubility : NMM-modified carriers have been reported to significantly increase the solubility of hydrophobic drugs.
- Controlled Release : The use of NMM allows for triggered release mechanisms based on environmental stimuli.
Organic Synthesis
In organic synthesis, this compound serves as a versatile intermediate. Its reactivity with nucleophiles allows for the formation of various derivatives that are useful in synthesizing complex molecules.
- Michael Addition Reactions : NMM participates in Michael addition reactions with nucleophiles, facilitating the synthesis of diverse compounds.
- Cycloaddition Reactions : The compound can also undergo cycloaddition reactions, expanding its utility in synthetic pathways.
Data Table: Synthetic Applications of this compound
Mechanism of Action
N-Methylmaleimide exerts its effects primarily through its reactivity with nucleophiles. The compound’s electron-deficient double bond makes it highly reactive towards nucleophiles, such as thiols and amines. This reactivity allows it to form stable covalent bonds with these nucleophiles, leading to the modification of biomolecules and other compounds.
Molecular Targets and Pathways:
Thiol Groups: Reacts with thiol groups in proteins and peptides, leading to the formation of thioether bonds.
Amines: Can also react with amine groups, although this is less common compared to thiol reactions.
Comparison with Similar Compounds
N-Methylmaleimide vs. N-Aryl Maleimides
- Asymmetric Conjugate Additions: In organocatalytic reactions, NMM (10f) and non-aryl maleimides (e.g., 10g) exhibit near-quantitative yields (98–99%) but lower enantioselectivity (73–78% ee) compared to N-aryl maleimides (e.g., 10d, 10e), which achieve 81–78% ee under similar conditions .
- Cycloadditions: NMM reacts with imino esters (e.g., 8a, 8b) to form cycloadducts with moderate yields (45–69%), while N-phenylmaleimide (9b) yields products with higher enantiomeric ratios (er = 93:7–98:2) .
This compound vs. Simple Maleimide
- Simple maleimide (10g) shows slightly reduced enantioselectivity (73% ee) compared to NMM (78% ee) in asymmetric additions, highlighting the electronic influence of the N-methyl group .
Table 1: Reaction Outcomes of Maleimides in Asymmetric Additions and Cycloadditions
Thiol Alkylation Efficiency
This compound vs. N-Ethylmaleimide (NEM)
- Reversibility : NEM reacts rapidly with thiols via Michael addition but is reversible, necessitating excess reagent. In contrast, NMM’s reactions are less studied for reversibility but are similarly concentration-dependent .
- Blocking Efficiency : At 1 mM, NMM fails to fully block thiols in high-glutathione (GSH) environments (>1 mM), whereas NEM’s higher reactivity ensures more complete thiol modification under similar conditions .
Table 2: Thiol Alkylation Performance
Compound | Optimal Concentration | Key Limitation | Reference |
---|---|---|---|
NMM | 1 mM | Incomplete blocking in high-GSH systems | |
NEM | 5–10 mM | Reversible reaction |
Substrate Specificity in Enzymes
- OYERo2a Enzyme Kinetics : NMM serves as a substrate for Old Yellow Enzymes (OYE), with wild-type OYERo2a showing a catalytic efficiency (kcat/Km) of 4600–5700 mM⁻¹s⁻¹. Mutants like C25S exhibit doubled kcat (147 U mg⁻¹) but tripled Km (30 µM), reducing overall efficiency .
- Cofactor Dependence: NMM’s reduction by OYERo2a is more efficient with NADPH than the synthetic cofactor BNAH, unlike N-ethylmaleimide derivatives, which show cofactor-agnostic activity .
Key Research Findings and Contradictions
- Thiol Blocking : While NMM is used interchangeably with NEM in biochemical assays, highlights its inferior blocking efficiency at 1 mM, urging caution in high-thiol environments .
- Electronic Effects : Computational studies reveal that NMM’s syn preference in Diels-Alder reactions stems from diene distortion energies (5.0 kcal/mol difference), unlike N-phenylmaleimide, where electronic effects dominate .
Biological Activity
N-Methylmaleimide (NMM) is a cyclic imide derivative that has garnered attention for its diverse biological activities. This article explores its synthesis, biological properties, and potential applications in various fields, particularly in medicinal chemistry.
Chemical Structure and Synthesis
This compound is characterized by its cyclic structure, which includes a maleimide moiety with a methyl group attached to the nitrogen atom. This modification enhances its solubility and biological activity. The synthesis typically involves the reaction of maleic anhydride with methylamine, leading to the formation of NMM in good yields.
Antimicrobial Activity
This compound exhibits significant antimicrobial properties. A study highlighted its antibacterial activity against Escherichia coli, demonstrating the compound's potential as an antimicrobial agent . Additionally, NMM has shown promising antifungal activity against various fungal pathogens, including Sclerotinia sclerotiorum. In a series of tests, NMM derivatives demonstrated EC50 values lower than those of conventional fungicides, indicating superior efficacy .
Compound | EC50 (µg/mL) | Activity |
---|---|---|
N-(3,5-Dichlorophenyl)-3,4-dichloromaleimide | 1.11 | Antifungal |
N-octyl-3-methylmaleimide | 1.01 | Antifungal |
Dicloran | 1.72 | Reference fungicide |
Antitumor Activity
Research indicates that this compound can enhance the cytotoxic activity of conventional chemotherapeutics like doxorubicin. In vitro studies have shown that NMM can inhibit cell proliferation in breast cancer cells more effectively when used in combination with doxorubicin, leading to a synergistic effect . The relative fold increase (RF) in cytotoxicity was notably higher for this compound compared to other derivatives.
Compound | RF Value | Concentration (mM) |
---|---|---|
This compound | 2.77 | 2.4 |
Reference Compound | 1.50 | 32.6 |
The mechanism behind the biological activity of this compound is attributed to its ability to interact with biological membranes and proteins due to its hydrophobic nature and electrophilic characteristics. This interaction facilitates the inhibition of drug efflux pumps such as P-glycoprotein (P-gp), which is crucial in multidrug resistance (MDR) observed in cancer cells .
Case Studies and Research Findings
- Antifungal Evaluation : A comprehensive study synthesized various maleimides, including this compound derivatives, and evaluated their antifungal activity against Sclerotinia sclerotiorum. The results indicated that certain derivatives were significantly more potent than established fungicides, suggesting their potential as lead compounds for new antifungal agents .
- Combination Therapy : In another investigation focusing on breast cancer cells, this compound was tested alongside doxorubicin. The findings revealed a marked increase in cytotoxicity when these compounds were used together, underscoring the potential for developing combination therapies that enhance treatment efficacy while minimizing side effects .
- Antibacterial Properties : Research conducted on various maleimides demonstrated that this compound exhibited notable antibacterial properties against E. coli, indicating its broader applicability in treating bacterial infections .
Q & A
Basic Research Questions
Q. How can the formation of Diels-Alder (DA) adducts with N-Methylmaleimide be monitored and quantified experimentally?
-
Methodological Answer: Use ¹H NMR spectroscopy to track DA adduct formation by observing proton signals from the furan (e.g., f1, f4) and maleimide (m2) moieties. Integrate peaks corresponding to starting materials (f1, m2) and adducts (f1’, m2’) to calculate conversion rates via equations such as:
Ensure solvent compatibility (e.g., D₂O or DMSO) and controlled temperatures (e.g., 40–65°C) to avoid side reactions .
Q. What are the optimal conditions for synthesizing DA adducts with this compound?
- Methodological Answer: Employ a molar excess of this compound (e.g., 20:1 ratio to diene) in polar solvents like DMSO or ethanol. Catalytic systems such as PPh₃AuNTf₂ (2 mol%) enhance reaction rates and endo-selectivity. Monitor reaction progress over 3–72 hours at 40–120°C depending on diene reactivity .
Q. How should this compound-containing waste be handled in alignment with green chemistry principles?
- Methodological Answer: Filtrates containing unreacted this compound can be reused in subsequent reactions by adding only one fresh equivalent. For disposal, use labeled aqueous waste containers due to the compound’s potential toxicity. Prioritize reactions with high atom economy (e.g., DA reactions) to minimize waste .
Advanced Research Questions
Q. Why does this compound exhibit irreversible Michael addition compared to other maleimides?
- Methodological Answer: DFT calculations reveal that this compound’s Michael addition with thiols (e.g., methyl cysteinate) is highly exergonic (ΔG° = -10.6 kcal/mol), favoring irreversible product formation. In contrast, less electron-deficient maleimides (e.g., cyclopentadienyl derivatives) show weaker thermodynamic driving forces (ΔG° = -2.4 kcal/mol), leading to equilibrium states. Validate experimentally via kinetic studies and NMR monitoring .
Q. How does steric hindrance influence this compound’s reactivity in organometallic systems?
- Methodological Answer: Steric bulk from substituents (e.g., Cp* in Rh complexes) can block dienophile access, preventing cycloaddition. For example, no DA adducts formed between [(Cp*)Rh(bpy)Cl]⁺ and this compound due to Cp* methyl crowding. Use DFT modeling to analyze interaction energies (e.g., maleimide C=O···H-OH bonds at 1.68 Å) and isotopic labeling (e.g., deuterium exchange) to probe steric effects .
Q. How can computational methods predict this compound’s selectivity in thiol-Michael reactions?
- Methodological Answer: Perform density functional theory (DFT) calculations to compare transition-state energies for competing pathways. For example, calculate Gibbs free energy (ΔG°) for thiolate attack on maleimide carbonyl vs. vinyl groups. Pair computational results with experimental kinetic data (e.g., rate constants from stopped-flow spectroscopy) to validate selectivity trends .
Q. Data Contradiction Analysis
Q. How to resolve discrepancies in emission intensity reports for this compound?
- Methodological Answer: Emission properties depend on the energy gap (ΔE) between n→π* and π→π* states. If conflicting data arise, verify solvent polarity, concentration, and excitation wavelengths. For example, a ΔE of 5400 cm⁻¹ correlates with weak emission in this compound, while succinimide (ΔE = 400 cm⁻¹) shows stronger fluorescence. Replicate experiments under identical conditions and use time-resolved spectroscopy to resolve ambiguities .
Q. Why do some catalytic systems fail to activate this compound in cross-coupling reactions?
- Methodological Answer: Evaluate ligand electronic effects. For Pd-catalyzed Stille couplings, electron-deficient ligands (e.g., Ph₂PfcC(O)PPh₂ ) enhance catalytic activity by stabilizing Pd(0)-maleimide adducts. If reactivity is low, screen ligands with stronger π-acceptor character and confirm maleimide coordination via X-ray crystallography or NMR .
Properties
IUPAC Name |
1-methylpyrrole-2,5-dione | |
---|---|---|
Source | PubChem | |
URL | https://pubchem.ncbi.nlm.nih.gov | |
Description | Data deposited in or computed by PubChem | |
InChI |
InChI=1S/C5H5NO2/c1-6-4(7)2-3-5(6)8/h2-3H,1H3 | |
Source | PubChem | |
URL | https://pubchem.ncbi.nlm.nih.gov | |
Description | Data deposited in or computed by PubChem | |
InChI Key |
SEEYREPSKCQBBF-UHFFFAOYSA-N | |
Source | PubChem | |
URL | https://pubchem.ncbi.nlm.nih.gov | |
Description | Data deposited in or computed by PubChem | |
Canonical SMILES |
CN1C(=O)C=CC1=O | |
Source | PubChem | |
URL | https://pubchem.ncbi.nlm.nih.gov | |
Description | Data deposited in or computed by PubChem | |
Molecular Formula |
C5H5NO2 | |
Source | PubChem | |
URL | https://pubchem.ncbi.nlm.nih.gov | |
Description | Data deposited in or computed by PubChem | |
DSSTOX Substance ID |
DTXSID30239240 | |
Record name | Maleimide, N-methyl- | |
Source | EPA DSSTox | |
URL | https://comptox.epa.gov/dashboard/DTXSID30239240 | |
Description | DSSTox provides a high quality public chemistry resource for supporting improved predictive toxicology. | |
Molecular Weight |
111.10 g/mol | |
Source | PubChem | |
URL | https://pubchem.ncbi.nlm.nih.gov | |
Description | Data deposited in or computed by PubChem | |
CAS No. |
930-88-1 | |
Record name | N-Methylmaleimide | |
Source | CAS Common Chemistry | |
URL | https://commonchemistry.cas.org/detail?cas_rn=930-88-1 | |
Description | CAS Common Chemistry is an open community resource for accessing chemical information. Nearly 500,000 chemical substances from CAS REGISTRY cover areas of community interest, including common and frequently regulated chemicals, and those relevant to high school and undergraduate chemistry classes. This chemical information, curated by our expert scientists, is provided in alignment with our mission as a division of the American Chemical Society. | |
Explanation | The data from CAS Common Chemistry is provided under a CC-BY-NC 4.0 license, unless otherwise stated. | |
Record name | N-Methylmaleimide | |
Source | ChemIDplus | |
URL | https://pubchem.ncbi.nlm.nih.gov/substance/?source=chemidplus&sourceid=0000930881 | |
Description | ChemIDplus is a free, web search system that provides access to the structure and nomenclature authority files used for the identification of chemical substances cited in National Library of Medicine (NLM) databases, including the TOXNET system. | |
Record name | N-Methylmaleimide | |
Source | DTP/NCI | |
URL | https://dtp.cancer.gov/dtpstandard/servlet/dwindex?searchtype=NSC&outputformat=html&searchlist=57594 | |
Description | The NCI Development Therapeutics Program (DTP) provides services and resources to the academic and private-sector research communities worldwide to facilitate the discovery and development of new cancer therapeutic agents. | |
Explanation | Unless otherwise indicated, all text within NCI products is free of copyright and may be reused without our permission. Credit the National Cancer Institute as the source. | |
Record name | Maleimide, N-methyl- | |
Source | EPA DSSTox | |
URL | https://comptox.epa.gov/dashboard/DTXSID30239240 | |
Description | DSSTox provides a high quality public chemistry resource for supporting improved predictive toxicology. | |
Record name | N-methylmaleimide | |
Source | European Chemicals Agency (ECHA) | |
URL | https://echa.europa.eu/substance-information/-/substanceinfo/100.012.024 | |
Description | The European Chemicals Agency (ECHA) is an agency of the European Union which is the driving force among regulatory authorities in implementing the EU's groundbreaking chemicals legislation for the benefit of human health and the environment as well as for innovation and competitiveness. | |
Explanation | Use of the information, documents and data from the ECHA website is subject to the terms and conditions of this Legal Notice, and subject to other binding limitations provided for under applicable law, the information, documents and data made available on the ECHA website may be reproduced, distributed and/or used, totally or in part, for non-commercial purposes provided that ECHA is acknowledged as the source: "Source: European Chemicals Agency, http://echa.europa.eu/". Such acknowledgement must be included in each copy of the material. ECHA permits and encourages organisations and individuals to create links to the ECHA website under the following cumulative conditions: Links can only be made to webpages that provide a link to the Legal Notice page. | |
Record name | N-METHYLMALEIMIDE | |
Source | FDA Global Substance Registration System (GSRS) | |
URL | https://gsrs.ncats.nih.gov/ginas/app/beta/substances/P0TFZ8R21Y | |
Description | The FDA Global Substance Registration System (GSRS) enables the efficient and accurate exchange of information on what substances are in regulated products. Instead of relying on names, which vary across regulatory domains, countries, and regions, the GSRS knowledge base makes it possible for substances to be defined by standardized, scientific descriptions. | |
Explanation | Unless otherwise noted, the contents of the FDA website (www.fda.gov), both text and graphics, are not copyrighted. They are in the public domain and may be republished, reprinted and otherwise used freely by anyone without the need to obtain permission from FDA. Credit to the U.S. Food and Drug Administration as the source is appreciated but not required. | |
Retrosynthesis Analysis
AI-Powered Synthesis Planning: Our tool employs the Template_relevance Pistachio, Template_relevance Bkms_metabolic, Template_relevance Pistachio_ringbreaker, Template_relevance Reaxys, Template_relevance Reaxys_biocatalysis model, leveraging a vast database of chemical reactions to predict feasible synthetic routes.
One-Step Synthesis Focus: Specifically designed for one-step synthesis, it provides concise and direct routes for your target compounds, streamlining the synthesis process.
Accurate Predictions: Utilizing the extensive PISTACHIO, BKMS_METABOLIC, PISTACHIO_RINGBREAKER, REAXYS, REAXYS_BIOCATALYSIS database, our tool offers high-accuracy predictions, reflecting the latest in chemical research and data.
Strategy Settings
Precursor scoring | Relevance Heuristic |
---|---|
Min. plausibility | 0.01 |
Model | Template_relevance |
Template Set | Pistachio/Bkms_metabolic/Pistachio_ringbreaker/Reaxys/Reaxys_biocatalysis |
Top-N result to add to graph | 6 |
Feasible Synthetic Routes
Disclaimer and Information on In-Vitro Research Products
Please be aware that all articles and product information presented on BenchChem are intended solely for informational purposes. The products available for purchase on BenchChem are specifically designed for in-vitro studies, which are conducted outside of living organisms. In-vitro studies, derived from the Latin term "in glass," involve experiments performed in controlled laboratory settings using cells or tissues. It is important to note that these products are not categorized as medicines or drugs, and they have not received approval from the FDA for the prevention, treatment, or cure of any medical condition, ailment, or disease. We must emphasize that any form of bodily introduction of these products into humans or animals is strictly prohibited by law. It is essential to adhere to these guidelines to ensure compliance with legal and ethical standards in research and experimentation.