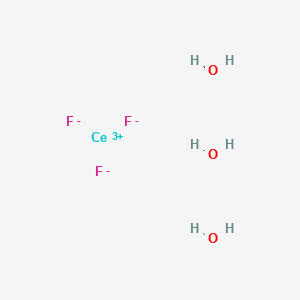
Cerium(III) fluoride trihydrate
- Click on QUICK INQUIRY to receive a quote from our team of experts.
- With the quality product at a COMPETITIVE price, you can focus more on your research.
Overview
Description
Cerium(III) fluoride trihydrate, with the chemical formula CeF₃·3H₂O, is an ionic compound consisting of cerium, a rare earth metal, and fluorine. This compound is known for its unique properties and applications in various fields, including chemistry, biology, medicine, and industry. Cerium(III) fluoride appears as a mineral in the form of fluocerite-(Ce), which is a rare mineral species related mainly to pegmatites and rarely to oxidation zones of some polymetallic ore deposits .
Preparation Methods
Synthetic Routes and Reaction Conditions: Cerium(III) fluoride trihydrate can be synthesized through several methods, including:
Direct Precipitation: This involves dissolving cerium nitrate hexahydrate in water and adding hydrofluoric acid to precipitate this compound.
Hydrothermal Synthesis: This method involves reacting cerium oxide with hydrofluoric acid under high temperature and pressure conditions.
Sol-Gel Method: This involves the hydrolysis and polycondensation of cerium alkoxides in the presence of hydrofluoric acid.
Industrial Production Methods: Industrial production of this compound typically involves the reaction of cerium oxide with hydrofluoric acid at elevated temperatures. The resulting product is then purified and crystallized to obtain the trihydrate form .
Chemical Reactions Analysis
Types of Reactions: Cerium(III) fluoride trihydrate undergoes various types of chemical reactions, including:
Oxidation: Cerium(III) fluoride can be oxidized to cerium(IV) fluoride using strong oxidizing agents such as fluorine gas.
Reduction: Cerium(IV) fluoride can be reduced back to cerium(III) fluoride using reducing agents like hydrogen gas.
Substitution: Cerium(III) fluoride can react with other halogens to form cerium(III) chloride, cerium(III) bromide, and cerium(III) iodide.
Common Reagents and Conditions:
Oxidation: Fluorine gas at elevated temperatures.
Reduction: Hydrogen gas at high temperatures.
Substitution: Hydrogen halides (HCl, HBr, HI) at room temperature.
Major Products Formed:
Oxidation: Cerium(IV) fluoride.
Reduction: Cerium(III) fluoride.
Substitution: Cerium(III) chloride, cerium(III) bromide, cerium(III) iodide.
Scientific Research Applications
Cerium(III) fluoride trihydrate has a wide range of applications in scientific research:
Chemistry: Used as a catalyst in various chemical reactions and as a precursor for the synthesis of other cerium compounds.
Biology: Investigated for its potential use in biological imaging and as a contrast agent in medical imaging techniques.
Mechanism of Action
The mechanism by which cerium(III) fluoride trihydrate exerts its effects involves several molecular targets and pathways:
Antioxidant Properties: Cerium(III) fluoride nanoparticles can scavenge reactive oxygen species (ROS) and prevent oxidative damage to cells. This is achieved through the redox cycling between cerium(III) and cerium(IV) states.
Radiation Protection: Cerium(III) fluoride nanoparticles can protect healthy cells from radiation-induced damage by reducing the formation of hydrogen peroxide and hydroxyl radicals.
Comparison with Similar Compounds
Cerium(III) fluoride trihydrate can be compared with other similar compounds, such as:
Lanthanum(III) fluoride (LaF₃): Similar in structure and properties, but cerium(III) fluoride has a higher refractive index and better optical properties.
Neodymium(III) fluoride (NdF₃): Similar in chemical behavior, but cerium(III) fluoride is more stable and less hygroscopic.
Praseodymium(III) fluoride (PrF₃): Similar in reactivity, but cerium(III) fluoride has better antioxidant properties and is more effective in radiation protection
This compound stands out due to its unique combination of chemical stability, optical properties, and biological activity, making it a valuable compound in various scientific and industrial applications.
Properties
Molecular Formula |
CeF3H6O3 |
---|---|
Molecular Weight |
251.157 g/mol |
IUPAC Name |
cerium(3+);trifluoride;trihydrate |
InChI |
InChI=1S/Ce.3FH.3H2O/h;3*1H;3*1H2/q+3;;;;;;/p-3 |
InChI Key |
CBRINXJIESQMBP-UHFFFAOYSA-K |
Canonical SMILES |
O.O.O.[F-].[F-].[F-].[Ce+3] |
Origin of Product |
United States |
Disclaimer and Information on In-Vitro Research Products
Please be aware that all articles and product information presented on BenchChem are intended solely for informational purposes. The products available for purchase on BenchChem are specifically designed for in-vitro studies, which are conducted outside of living organisms. In-vitro studies, derived from the Latin term "in glass," involve experiments performed in controlled laboratory settings using cells or tissues. It is important to note that these products are not categorized as medicines or drugs, and they have not received approval from the FDA for the prevention, treatment, or cure of any medical condition, ailment, or disease. We must emphasize that any form of bodily introduction of these products into humans or animals is strictly prohibited by law. It is essential to adhere to these guidelines to ensure compliance with legal and ethical standards in research and experimentation.