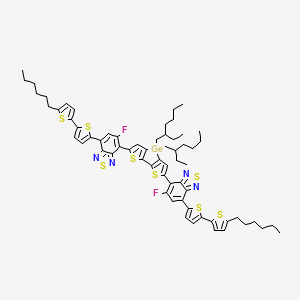
DTG(FBTTh2)
- Click on QUICK INQUIRY to receive a quote from our team of experts.
- With the quality product at a COMPETITIVE price, you can focus more on your research.
Overview
Description
DTG(FBTTh2) is a semiconducting material used primarily in organic photovoltaics. Its full chemical name is 7,7’-[4,4-bis(2-ethylhexyl)-4H-germolo[3,2-b:4,5-b’]dithiophene-2,6-diyl]bis[6-fluoro-4-(5’-hexyl-[2,2’-bithiophen]-5-yl)benzo[c][1,2,5]thiadiazole]. This compound is known for its low band-gap properties, making it an efficient donor material in bulk heterojunction solar cells .
Preparation Methods
Synthetic Routes and Reaction Conditions
The synthesis of DTG(FBTTh2) involves multiple steps, starting with the preparation of the germolo[3,2-b:4,5-b’]dithiophene core. This core is then functionalized with fluoro-bithiophene and benzo[c][1,2,5]thiadiazole units. The reaction conditions typically involve the use of palladium-catalyzed cross-coupling reactions, such as Suzuki or Stille coupling, under inert atmospheres like nitrogen or argon .
Industrial Production Methods
Industrial production of DTG(FBTTh2) follows similar synthetic routes but on a larger scale. The process involves stringent control of reaction conditions to ensure high purity and yield. Solvents like chloroform and chlorobenzene are commonly used for processing .
Chemical Reactions Analysis
Types of Reactions
DTG(FBTTh2) undergoes various chemical reactions, including:
Oxidation: Typically involves reagents like hydrogen peroxide or oxygen under controlled conditions.
Reduction: Can be achieved using reducing agents such as lithium aluminum hydride.
Substitution: Often involves halogenation or nitration reactions using reagents like bromine or nitric acid.
Common Reagents and Conditions
Oxidation: Hydrogen peroxide, oxygen.
Reduction: Lithium aluminum hydride.
Substitution: Bromine, nitric acid.
Major Products
The major products formed from these reactions depend on the specific reagents and conditions used. For example, oxidation may yield various oxidized derivatives, while substitution reactions can introduce different functional groups into the molecule .
Scientific Research Applications
DTG(FBTTh2) has a wide range of applications in scientific research:
Chemistry: Used as a donor material in organic photovoltaics and organic field-effect transistors.
Medicine: Research is ongoing into its use in medical
Properties
Molecular Formula |
C64H72F2GeN4S8 |
---|---|
Molecular Weight |
1264.4 g/mol |
IUPAC Name |
4-[7,7-bis(2-ethylhexyl)-10-[5-fluoro-7-[5-(5-hexylthiophen-2-yl)thiophen-2-yl]-2,1,3-benzothiadiazol-4-yl]-3,11-dithia-7-germatricyclo[6.3.0.02,6]undeca-1(8),2(6),4,9-tetraen-4-yl]-5-fluoro-7-[5-(5-hexylthiophen-2-yl)thiophen-2-yl]-2,1,3-benzothiadiazole |
InChI |
InChI=1S/C64H72F2GeN4S8/c1-7-13-17-19-23-41-25-27-51(72-41)53-31-29-49(74-53)43-33-45(65)57(61-59(43)68-78-70-61)55-35-47-63(76-55)64-48(67(47,37-39(11-5)21-15-9-3)38-40(12-6)22-16-10-4)36-56(77-64)58-46(66)34-44(60-62(58)71-79-69-60)50-30-32-54(75-50)52-28-26-42(73-52)24-20-18-14-8-2/h25-36,39-40H,7-24,37-38H2,1-6H3 |
InChI Key |
VIMLQZLOFLWSCV-UHFFFAOYSA-N |
Canonical SMILES |
CCCCCCC1=CC=C(S1)C2=CC=C(S2)C3=CC(=C(C4=NSN=C34)C5=CC6=C(S5)C7=C([Ge]6(CC(CC)CCCC)CC(CC)CCCC)C=C(S7)C8=C(C=C(C9=NSN=C89)C1=CC=C(S1)C1=CC=C(S1)CCCCCC)F)F |
Origin of Product |
United States |
Disclaimer and Information on In-Vitro Research Products
Please be aware that all articles and product information presented on BenchChem are intended solely for informational purposes. The products available for purchase on BenchChem are specifically designed for in-vitro studies, which are conducted outside of living organisms. In-vitro studies, derived from the Latin term "in glass," involve experiments performed in controlled laboratory settings using cells or tissues. It is important to note that these products are not categorized as medicines or drugs, and they have not received approval from the FDA for the prevention, treatment, or cure of any medical condition, ailment, or disease. We must emphasize that any form of bodily introduction of these products into humans or animals is strictly prohibited by law. It is essential to adhere to these guidelines to ensure compliance with legal and ethical standards in research and experimentation.