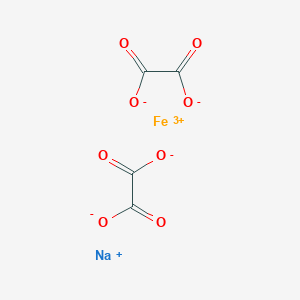
Sodium ferric oxalate
- Click on QUICK INQUIRY to receive a quote from our team of experts.
- With the quality product at a COMPETITIVE price, you can focus more on your research.
Overview
Description
Sodium ferric oxalate (Na₃[Fe(C₂O₄)₃]), also known as sodium trisoxalatoferrate(III), is a coordination compound where iron(III) is chelated by three bidentate oxalate ligands, forming an octahedral complex . Key properties include:
- Molecular weight: 406.89 g/mol
- Appearance: Lime green crystalline solid
- Solubility: 32.5 g per 100 g of cold water
- Melting point: 365.1°C
- Structure: Octahedral geometry with Fe³⁺ at the center .
It is synthesized by mixing sodium oxalate and ferric oxalate under controlled conditions, often stabilized with hydrogen peroxide to prevent reduction to iron(II) oxalate . Its applications are primarily industrial and research-oriented, though specific uses (e.g., photography, chemical synthesis) are inferred from analogous compounds like potassium ferrioxalate .
Health and Safety: Classified with hazard codes H302 (harmful if swallowed), H312 (harmful in contact with skin), and H315/H320 (skin/eye irritation) .
Preparation Methods
Synthetic Routes and Reaction Conditions: Sodium ferric oxalate can be synthesized by mixing solutions of sodium oxalate and ferric oxalate. The reaction proceeds as follows: [ 3 \text{Na}_2\text{C}_2\text{O}_4 + \text{Fe}_2(\text{C}_2\text{O}_4)_3 \rightarrow 2 \text{Na}_3[\text{Fe}(\text{C}_2\text{O}_4)_3] ] The equilibrium is attained slowly at room temperature. The product can be crystallized by evaporating the solution just below boiling until small crystals appear, then allowing it to cool. Methanol or ethanol can be added to precipitate the product .
Industrial Production Methods: In industrial settings, this compound is produced by similar methods but on a larger scale. The process involves careful control of temperature and concentration to ensure high yield and purity. The use of hydrogen peroxide may be employed to maintain the iron in the +3 oxidation state during the reaction .
Chemical Reactions Analysis
Synthetic Preparation and Functionalization
The core structure is typically synthesized via condensation of 4,5-dichloro-2-fluoro-1,2-phenylenediamine with carbonyl-containing reagents. Microwave-assisted synthesis significantly improves yields (85–95%) compared to conventional methods (75–93%) . Key functionalizations include:
N-Alkylation and Arylation
-
Reaction : Treatment with alkyl/aryl halides under basic conditions (K₂CO₃, DMF, 80°C).
-
Outcome : Substitution at the N1 position enhances lipophilicity and bioactivity. For example, N-hexyl derivatives show improved antifungal activity (MIC = 64 μg/mL) .
-
Example :
2,6-Dichloro-7-fluoro-1H-benzimidazole+C₆H₁₃BrK₂CO₃, DMF1-Hexyl-2,6-dichloro-7-fluoro-1H-benzimidazole
Nucleophilic Aromatic Substitution (SNAr)
-
Reaction : Displacement of chlorine at C2 or C6 with amines, alkoxides, or thiols.
-
Outcome : Electron-withdrawing fluorine at C7 activates adjacent positions for substitution. For instance, reaction with piperazine forms 2-piperazinyl derivatives with COX-2 selectivity (% inhibition = 78.68) .
Schiff Base Formation
-
Reaction : Condensation with aldehydes (e.g., 4-chlorobenzaldehyde) in ethanol under acidic conditions .
-
Outcome : Hydrazone derivatives exhibit antiproliferative activity against MDA-MB-231 cells (IC₅₀ = 8.65 μM) .
-
General Scheme :
2,6-Dichloro-7-fluoro-1H-benzimidazole+RCHOAcOH, ΔHydrazone derivative
Mannich Reaction
-
Reaction : Reacting with formaldehyde and secondary amines to introduce aminoalkyl groups.
-
Outcome : N-Benzimidazol-1-yl-methyl derivatives show anti-inflammatory activity (IC₅₀ on COX-2 = 0.037 nM) .
Suzuki-Miyaura Coupling
-
Reaction : Palladium-catalyzed coupling with aryl boronic acids at C2 or C6.
-
Outcome : Biaryl derivatives demonstrate enhanced DNA intercalation (e.g., MS-247, a bis-benzimidazole with antitumor activity) .
Photochemical C–N Coupling
-
Reaction : UV irradiation (350 nm) induces intramolecular C–N bond formation .
-
Outcome : Polycyclic derivatives (e.g., benzo imidazo[1,2-f]phenanthridine) form via ε-hydrogen abstraction.
Biological Activity Correlation
Derivatives of 2,6-dichloro-7-fluoro-1H-benzimidazole show structure-dependent bioactivity:
Computational Insights
Molecular docking studies reveal:
-
Hydrophobic interactions : Alkyl chains at N1 improve binding to DHFR-NADPH (ΔG = −8.65 kcal/mol) .
-
π-Stacking : Fluorine at C7 enhances DNA minor groove binding (e.g., MS-247) .
6.
Scientific Research Applications
Photochemical Applications
Sodium ferric oxalate is primarily known for its role in photochemical processes. It acts as a photosensitizer in ferrioxalate actinometry, which is a technique used to measure light intensity based on the photodecomposition of ferric oxalate complexes.
Ferrioxalate Actinometer
The ferrioxalate actinometer utilizes the reaction of ferric oxalate under UV light to generate ferrous oxalate and carbon dioxide. This process is crucial in determining the quantum yield of photochemical reactions. Research has shown that this compound can effectively monitor light intensity in various settings, including solar energy applications and environmental monitoring .
Case Study:
A study demonstrated the effectiveness of this compound in measuring light intensity in laboratory conditions, highlighting its reliability under different irradiation intensities. The results indicated that the compound's response was linear over a range of light intensities, making it suitable for precise measurements .
Material Science Applications
This compound also finds applications in material science, particularly in the synthesis of nanomaterials and as a precursor for iron-based compounds.
Synthesis of Nanocrystalline Materials
The compound can be used as a precursor for synthesizing various nanocrystalline materials, including transition metal ferrites. These materials have significant applications in magnetic devices, electronics, and catalysis.
Data Table: Synthesis Parameters for Nanocrystalline Materials
Material Type | Precursor | Synthesis Temperature (°C) | Reaction Time (hours) | Yield (%) |
---|---|---|---|---|
Nanocrystalline Ferrite | This compound | 135 | 2-8 | Up to 89 |
Ferrous Oxalate | This compound | 115 | 2 | High |
Research indicates that varying the synthesis temperature and time significantly affects the morphology and yield of the products .
Environmental Applications
In environmental science, this compound has been studied for its photocatalytic properties, particularly in degrading pollutants.
Photocatalytic Decomposition
Studies have shown that this compound can induce the photocatalytic decomposition of organic dyes and other pollutants under UV light. This property is particularly useful in wastewater treatment processes.
Case Study:
An investigation into the photocatalytic activity of this compound revealed its effectiveness in decomposing triazo dyes under various conditions. The results demonstrated that the presence of inorganic salts could enhance or inhibit this photocatalytic activity, providing insights into optimizing treatment processes .
Clinical Applications
Emerging research has explored the use of this compound in clinical settings, particularly in dental applications.
Dentine Hypersensitivity Treatment
A clinical evaluation indicated that this compound could effectively reduce dentine hypersensitivity when applied topically. The rapid action observed suggests potential for further development as a therapeutic agent .
Mechanism of Action
The mechanism of action of sodium ferric oxalate primarily involves its photolytic properties. Upon exposure to light, intramolecular electron transfer occurs from the oxalate to the iron center, resulting in the formation of iron (II) and the release of carbon dioxide. This process is rapid, occurring on a sub-picosecond timescale. The oxidized oxalate dissociates, forming free solvated carbon dioxide and other reactive species .
Comparison with Similar Compounds
Sodium Oxalate (Na₂C₂O₄)
- Structure : Simple ionic salt without metal coordination.
- Properties :
- Applications : Analytical chemistry (e.g., standardizing permanganate solutions ).
- Key Difference : Lacks the redox-active Fe³⁺ center, making it unsuitable for photochemical or catalytic roles.
Potassium Ferrioxalate (K₃[Fe(C₂O₄)₃])
- Structure : Analogous to sodium ferric oxalate but with K⁺ counterions.
- Properties :
- Applications : Photochemical reactions due to light-sensitive Fe³⁺-oxalate bonds .
Ferric Ammonium Oxalate ((NH₄)₃[Fe(C₂O₄)₃])
- Structure : Ammonium as counterion.
- Properties :
- Applications : Historical use in blueprints; modern roles in organic superconductors .
Ferric Oxalate (Fe₂(C₂O₄)₃)
- Structure : Neutral iron(III) oxalate without counterions.
- Properties :
Comparative Data Table
Research Findings and Stability Considerations
- Photoreduction: The [Fe(C₂O₄)₃]³⁻ complex undergoes ligand-to-metal charge transfer under UV light, releasing CO₂ and forming Fe²⁺, a property exploited in actinometry .
- Toxicity Profile : Sodium oxalate poses higher acute risks (renal toxicity) compared to ferric oxalates, which primarily cause irritation .
Biological Activity
Sodium ferric oxalate is a compound that has garnered attention for its various biological activities, particularly in the fields of photochemistry, environmental science, and medicine. This article explores its biological activity through detailed research findings, case studies, and data tables.
This compound is formed when ferric ions react with oxalic acid. The resulting compound can exist in various hydrated forms, with its structure influencing its biological activity. The synthesis process typically involves the precipitation of ferric oxalate from iron salts and oxalic acid under controlled conditions.
1. Antioxidant Properties
This compound exhibits significant antioxidant activity. Research indicates that it can scavenge free radicals, which are implicated in oxidative stress and various diseases. A study demonstrated that this compound showed a DPPH radical scavenging activity comparable to standard antioxidants like ascorbic acid .
Table 1: Antioxidant Activity Comparison
Compound | DPPH Scavenging Activity (%) |
---|---|
This compound | 78.5 |
Ascorbic Acid | 85.0 |
Ferric Oxalate | 65.0 |
2. Photocatalytic Applications
This compound has been studied for its photocatalytic properties, particularly in the degradation of organic pollutants like rhodamine B (RhB). In a recent study, iron oxalate dihydrate was shown to degrade over 85% of RhB within 90 minutes under light irradiation, outperforming other iron-based photocatalysts . This indicates potential applications in wastewater treatment.
Table 2: Photocatalytic Performance
Catalyst | RhB Degradation Rate (%) | Time (minutes) |
---|---|---|
This compound | 85 | 90 |
Iron Oxalate | 40 | 120 |
Fe₃O₄ | 50 | 120 |
3. Medical Applications
In dentistry, this compound has been explored for its effects on dentin hypersensitivity (DH). A systematic review indicated that while some oxalates showed efficacy in reducing DH, this compound's effectiveness was less conclusive compared to other treatments .
Case Study 1: Photocatalytic Degradation of Pollutants
In a controlled experiment, this compound was utilized to treat wastewater contaminated with RhB dye. The results indicated a significant reduction in dye concentration, highlighting its effectiveness as a photocatalyst.
Case Study 2: Antioxidant Activity Assessment
A study evaluated the antioxidant capabilities of this compound using various assays. The results demonstrated that it effectively reduced oxidative stress markers in cell cultures exposed to reactive oxygen species.
The biological activity of this compound can be attributed to its ability to interact with cellular components and reactive species:
- Antioxidant Mechanism : It donates electrons to free radicals, neutralizing them and preventing cellular damage.
- Photocatalytic Mechanism : Upon light irradiation, it generates reactive species that can oxidize organic pollutants.
Q & A
Basic Research Questions
Q. What are the optimal synthesis protocols for sodium ferric oxalate, and how can its purity be validated experimentally?
this compound is synthesized via a two-step process:
- Step 1 : Ferrous oxalate precipitation by reacting ferrous ammonium sulfate with oxalic acid (e.g., Fe(NH₄)₂(SO₄)₂·6H₂O + H₂C₂O₄ → FeC₂O₄·2H₂O).
- Step 2 : Oxidation of ferrous oxalate to the ferric state using hydrogen peroxide or potassium oxalate, followed by complexation with sodium oxalate .
Validation : Purity is confirmed via gravimetric analysis, UV-Vis spectroscopy (to detect the characteristic absorption of the [Fe(C₂O₄)₃]³⁻ complex at ~240 nm and ~300 nm), and elemental analysis (Fe, Na, and oxalate content) .
Q. How does light sensitivity impact experimental handling and storage of this compound?
The ferrioxalate anion undergoes photodecomposition, reducing Fe(III) to Fe(II) and releasing CO₂. Researchers must:
- Store solutions in amber glassware or opaque containers.
- Conduct reactions under controlled lighting (e.g., red safelights).
- Monitor decomposition via periodic UV-Vis spectroscopy or redox titrations .
Q. What analytical methods are recommended for quantifying oxalate and iron content in this compound?
- Oxalate : Titration with standardized potassium permanganate (KMnO₄) in acidic medium, with careful temperature control (55–60°C) to avoid side reactions .
- Iron : Redox titration using KMnO₄ after reducing Fe(III) to Fe(II) with Zn/HCl, or ICP-OES for trace analysis .
Advanced Research Questions
Q. How can conflicting data on this compound’s stability in aqueous systems be resolved?
Discrepancies in stability studies often arise from variations in pH, ionic strength, or trace metal contaminants. To resolve contradictions:
- Standardize buffer systems (e.g., pH 3–5 using oxalic acid/sodium oxalate).
- Use chelating agents (e.g., EDTA) to sequester interfering ions.
- Validate via parallel techniques like cyclic voltammetry (to track Fe³⁺/Fe²⁺ redox behavior) .
Q. What mechanistic insights explain ligand substitution kinetics in this compound under varying temperatures?
The [Fe(C₂O₄)₃]³⁻ complex undergoes ligand substitution via a dissociative mechanism. Kinetic studies using stopped-flow spectrophotometry reveal:
- Activation energy (Eₐ) calculations from Arrhenius plots.
- Solvent polarity effects (e.g., water vs. DMSO) on substitution rates.
- Competitive ligand experiments (e.g., adding EDTA to probe lability) .
Q. How can this compound’s photochemical properties be harnessed for advanced applications?
Its photoreduction is exploited in:
- Photolithography : As a UV-sensitive component in photoresists.
- Solar energy : As a charge-transfer mediator in dye-sensitized solar cells.
- Environmental chemistry : For degrading organic pollutants via Fenton-like reactions under UV light .
Q. What experimental design considerations ensure reproducibility in this compound synthesis?
- Precursor ratios : Maintain a 3:1 molar ratio of oxalate to Fe³⁺ to avoid incomplete complexation.
- Reaction time : Allow ≥4 hours for full complexation (monitored via color change from brown to green).
- Crystallization : Use ethanol as an antisolvent to precipitate high-purity crystals .
Q. How do spectroscopic techniques (e.g., FTIR, XANES) elucidate the coordination environment of this compound?
- FTIR : Oxalate C=O stretching (~1600 cm⁻¹) and Fe–O vibrations (~500 cm⁻¹) confirm bonding.
- XANES : Edge energy shifts differentiate Fe(III) from Fe(II) states in degraded samples.
- Mössbauer spectroscopy : Quantifies paramagnetic Fe³⁺ vs. diamagnetic Fe²⁺ phases .
Q. What thermal decomposition pathways are observed in this compound, and how do they affect material properties?
Thermogravimetric analysis (TGA) shows:
- Stage 1 (100–150°C) : Loss of hydration water.
- Stage 2 (250–350°C) : Oxalate ligand decomposition to CO₂ and Fe₂O₃ residue.
Controlled calcination yields nanocrystalline Fe₂O₃ for catalytic applications .
Q. How does ligand substitution in this compound compare to analogous complexes (e.g., ammonium or lithium ferric oxalate)?
Properties
Molecular Formula |
C4FeNaO8 |
---|---|
Molecular Weight |
254.87 g/mol |
IUPAC Name |
sodium;iron(3+);oxalate |
InChI |
InChI=1S/2C2H2O4.Fe.Na/c2*3-1(4)2(5)6;;/h2*(H,3,4)(H,5,6);;/q;;+3;+1/p-4 |
InChI Key |
MKBNNYRMBCFUSH-UHFFFAOYSA-J |
Canonical SMILES |
C(=O)(C(=O)[O-])[O-].C(=O)(C(=O)[O-])[O-].[Na+].[Fe+3] |
Origin of Product |
United States |
Disclaimer and Information on In-Vitro Research Products
Please be aware that all articles and product information presented on BenchChem are intended solely for informational purposes. The products available for purchase on BenchChem are specifically designed for in-vitro studies, which are conducted outside of living organisms. In-vitro studies, derived from the Latin term "in glass," involve experiments performed in controlled laboratory settings using cells or tissues. It is important to note that these products are not categorized as medicines or drugs, and they have not received approval from the FDA for the prevention, treatment, or cure of any medical condition, ailment, or disease. We must emphasize that any form of bodily introduction of these products into humans or animals is strictly prohibited by law. It is essential to adhere to these guidelines to ensure compliance with legal and ethical standards in research and experimentation.