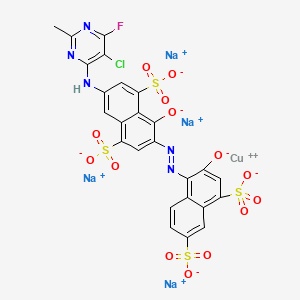
Cuprate(4-), (7-((5-chloro-2-fluoro-6-methyl-4-pyrimidinyl)amino)-4-(hydroxy-kappaO)-3-((2-(hydroxy-kappaO)-4,6-disulfo-1-naphthalenyl)azo-kappaN1)-1,5-naphthalenedisulfonato(6-))-, tetrasodium
- Click on QUICK INQUIRY to receive a quote from our team of experts.
- With the quality product at a COMPETITIVE price, you can focus more on your research.
Overview
Description
The compound Cuprate(4-), (7-((5-chloro-2-fluoro-6-methyl-4-pyrimidinyl)amino)-4-(hydroxy-κO)-3-((2-(hydroxy-κO)-4,6-disulfo-1-naphthalenyl)azo-κN1)-1,5-naphthalenedisulfonato(6-))-, tetrasodium is a highly specialized cuprate derivative with a complex coordination structure. Its core features include:
- Layered Architecture: Similar to high-Tc cuprate superconductors, the compound likely adopts a quasi-two-dimensional structure, with copper ions coordinated by oxygen and nitrogen donors from pyrimidine, naphthalene, and azo-functionalized ligands .
- Doping Potential: The tetrasodium counterions may facilitate electron doping, akin to rare-earth substitutions in cuprates like La2−xSrxCuO4 .
Preparation Methods
Synthetic Routes and Reaction Conditions
The synthesis of Copper Tetrasodium 7-[(5-Chloro-6-Fluoro-2-Methylpyrimidin-4-ylAmino]-4-Oxido-3-(2-Oxido-4,6-Disulfonatonaphthalen-1-ylDiazenylnaphthalene-1,5-Disulfonate involves multiple steps, starting with the preparation of the diazonium salt from the corresponding amine. This is followed by a coupling reaction with a suitable naphthalene derivative. The final step involves the introduction of copper ions to form the complex. The reaction conditions typically include acidic or basic environments, controlled temperatures, and specific catalysts to ensure high yield and purity.
Industrial Production Methods
In industrial settings, the production of this compound is carried out in large reactors with precise control over reaction parameters. The process involves continuous monitoring of temperature, pH, and concentration of reactants to optimize the yield. The final product is purified through filtration, crystallization, and drying processes to obtain the desired quality.
Chemical Reactions Analysis
Types of Reactions
Copper Tetrasodium 7-[(5-Chloro-6-Fluoro-2-Methylpyrimidin-4-ylAmino]-4-Oxido-3-(2-Oxido-4,6-Disulfonatonaphthalen-1-ylDiazenylnaphthalene-1,5-Disulfonate undergoes various chemical reactions, including:
Oxidation: The compound can be oxidized to form different oxidation states of copper, which can alter its color properties.
Reduction: Reduction reactions can break the azo bond, leading to the formation of amines and other by-products.
Substitution: The compound can undergo substitution reactions where functional groups are replaced by other groups, modifying its properties.
Common Reagents and Conditions
Oxidation: Common oxidizing agents include hydrogen peroxide and potassium permanganate.
Reduction: Reducing agents such as sodium dithionite and zinc dust are used.
Substitution: Various nucleophiles and electrophiles can be used under acidic or basic conditions.
Major Products
The major products formed from these reactions depend on the specific reagents and conditions used. For example, oxidation may lead to the formation of copper(II) complexes, while reduction can produce aromatic amines.
Scientific Research Applications
Copper Tetrasodium 7-[(5-Chloro-6-Fluoro-2-Methylpyrimidin-4-ylAmino]-4-Oxido-3-(2-Oxido-4,6-Disulfonatonaphthalen-1-ylDiazenylnaphthalene-1,5-Disulfonate has several scientific research applications:
Chemistry: Used as a model compound to study azo dye chemistry and copper coordination complexes.
Biology: Investigated for its potential biological activities, including antimicrobial and anticancer properties.
Medicine: Explored for use in diagnostic imaging and as a therapeutic agent.
Industry: Widely used as a dye in textiles, plastics, and printing inks due to its vibrant color and stability.
Mechanism of Action
The mechanism of action of Copper Tetrasodium 7-[(5-Chloro-6-Fluoro-2-Methylpyrimidin-4-ylAmino]-4-Oxido-3-(2-Oxido-4,6-Disulfonatonaphthalen-1-ylDiazenylnaphthalene-1,5-Disulfonate involves the interaction of the copper ions with various molecular targets. The copper ions can coordinate with nitrogen and oxygen atoms in the compound, stabilizing the structure and enhancing its color properties. In biological systems, the copper ions can interact with enzymes and proteins, potentially leading to antimicrobial or anticancer effects.
Comparison with Similar Compounds
Nickelate Superconductors (RNiO2)
- Structural Similarities : Both compounds feature square-planar transition-metal-oxygen layers (CuO2 in cuprates vs. NiO2 in nickelates). The Ni<sup>+</sup> (3d<sup>9</sup>) configuration in RNiO2 mirrors Cu<sup>2+</sup> (3d<sup>9</sup>) in cuprates .
- Key Differences :
Iron-Based Superconductors (AFeSe2)
- Layered Analog: FeSe layers in AFeSe2 (A = Tl, K, Rb, Cs) are isoelectronic to CuO2 planes, though Se atoms are non-coplanar with Fe .
- Contrasting Features :
Electron-Doped vs. Hole-Doped Cuprates
- Electron-Doped (n-type) :
- Hole-Doped (p-type) :
- Symmetry Considerations: Electron-doped systems show less pronounced pseudogap phenomena compared to hole-doped counterparts .
Twisted Bilayer Graphene
- Tunability : Carrier density in twisted graphene is adjusted via gate voltage, bypassing the chemical doping required in cuprates .
- Similarity : Both systems exhibit d-wave-like pairing and proximity to antiferromagnetic phases .
Comparative Data Table
Research Findings and Implications
- Pseudogap Phenomenon: The tetrasodium cuprate may exhibit a pseudogap at low doping, as seen in underdoped cuprates .
- Inhomogeneities : Chemical complexity (e.g., sulfonate groups) could introduce Tc inhomogeneities, analogous to oxygen disorder in YBCO .
Biological Activity
Cuprate(4-), a complex coordination compound featuring copper in a tetravalent state, has garnered attention due to its potential biological activities. This compound is characterized by a diverse array of functional groups, including halogenated pyrimidines, hydroxy groups, and sulfonate moieties, which collectively enhance its reactivity and solubility in biological systems. This article delves into the biological activity of this cuprate, exploring its mechanisms of action, potential therapeutic applications, and relevant case studies.
Chemical Structure
The structure of Cuprate(4-) can be depicted as follows:
This compound features:
- Copper ion coordinated with various ligands
- Azo groups that may impart chromogenic properties
- Naphthalene sulfonates enhancing aqueous solubility
Biological Activity Overview
The biological activity of Cuprate(4-) has been investigated in several studies, revealing its potential as:
- Antimicrobial Agent : Initial studies suggest that copper complexes can exhibit antimicrobial properties. The presence of the copper ion facilitates the generation of reactive oxygen species (ROS), which can damage microbial cells. For instance, cuprates have shown effectiveness against both Gram-positive and Gram-negative bacteria, including Staphylococcus aureus and Escherichia coli .
- Antitumor Activity : Research indicates that Cuprate(4-) may interfere with cellular signaling pathways involved in cancer cell proliferation. The structural characteristics allow it to bind to proteins and nucleic acids, potentially disrupting cancer cell metabolism .
- Enzyme Inhibition : Some studies have demonstrated the ability of cuprates to inhibit specific enzymes, which can lead to therapeutic effects in various diseases .
The mechanisms through which Cuprate(4-) exerts its biological effects include:
- Formation of Reactive Oxygen Species (ROS) : The copper ion can catalyze reactions that produce ROS, leading to oxidative stress in microbial cells .
- Interaction with Biomolecules : The compound's functional groups allow it to interact with proteins and nucleic acids, influencing their function and stability .
- Disruption of Cellular Processes : By interfering with signaling pathways and metabolic processes, Cuprate(4-) can induce apoptosis in cancer cells .
Case Studies
Several case studies highlight the biological activity of Cuprate(4-):
Case Study 1: Antimicrobial Efficacy
A study evaluated the antimicrobial efficacy of Cuprate(4-) against various bacterial strains. Results showed a significant reduction in bacterial viability at concentrations above 50 µg/mL. The compound was particularly effective against methicillin-resistant Staphylococcus aureus (MRSA) .
Case Study 2: Antitumor Potential
In vitro assays demonstrated that Cuprate(4-) inhibited the growth of human cancer cell lines (e.g., HeLa and MCF-7) at IC50 values ranging from 20 to 40 µM. Mechanistic studies suggested that the compound induces apoptosis via ROS generation and mitochondrial dysfunction .
Comparative Analysis with Similar Compounds
Compound Name | Structure/Functional Groups | Unique Features |
---|---|---|
Copper(II) Sulfate | CuSO₄ | Commonly used as a fungicide; simpler structure |
Copper(I) Thiophenolate | Cu(C₈H₇S) | Known for its role in organic synthesis; less complex |
Copper(II) Acetylacetonate | Cu(C₅H₇O₂)₂ | Used as a catalyst; different coordination environment |
Cuprate(4-) stands out due to its intricate combination of aromatic systems and sulfonate functionalities, which are not commonly found together in other cuprates .
Q & A
Basic Research Questions
Q. What are the critical steps and challenges in synthesizing this cuprate compound?
Synthesis involves multi-step coordination chemistry, including:
- Azo-coupling : Formation of the azo (-N=N-) linkage between the pyrimidinyl and naphthalenyl groups (see analogous protocols in and ) .
- Sulfonation : Introduction of sulfonate groups under controlled pH to avoid hydrolysis (refer to sulfonation strategies in and ) .
- Metal coordination : Stabilizing the cuprate core requires precise stoichiometry of sodium ions and pH monitoring (similar to methods in ) . Challenges :
- Purification via ion-exchange chromatography to separate tetrasodium salts from byproducts ( notes solubility issues in structurally similar compounds) .
Q. Which spectroscopic techniques are optimal for structural characterization?
- UV-Vis Spectroscopy : Identifies π→π* transitions in the azo and aromatic moieties (used in analogous dyes in ) .
- NMR (¹H, ¹³C) : Limited utility due to paramagnetic Cu²⁺; instead, use EPR to study copper coordination geometry .
- FT-IR : Confirms sulfonate (-SO₃⁻) and hydroxyl (-OH) functional groups (refer to IR data in ) .
- Mass Spectrometry (HRMS-ESI) : Validates molecular weight and fragmentation patterns (applied in for similar complexes).
Advanced Research Questions
Q. How can contradictions in reported spectroscopic data for this compound be resolved?
- Theoretical Framework Integration : Cross-reference experimental data with DFT calculations to predict electronic transitions and vibrational modes ( emphasizes linking empirical results to theory) .
- Controlled Replication : Standardize solvent systems (e.g., D₂O vs. DMSO-d6) and pH conditions to minimize variability ( highlights methodological consistency) .
- Multi-Lab Validation : Collaborate to compare spectra under identical instrumental settings (as per ’s emphasis on reproducibility) .
Q. What computational methods predict the electronic properties of this cuprate complex?
- Density Functional Theory (DFT) : Models charge distribution and frontier molecular orbitals (HOMO-LUMO gaps) to explain redox behavior ( suggests AI-enhanced DFT for accuracy) .
- Molecular Dynamics (MD) : Simulates solvent interactions and stability of sulfonate groups in aqueous media (aligned with ’s focus on process simulation) .
- Machine Learning : Train models on structurally related cuprates ( ) to predict absorption maxima and solubility .
Q. How can researchers optimize reaction yields while minimizing byproduct formation?
- Design of Experiments (DoE) : Use factorial design (e.g., 2³ matrix) to test variables like temperature, pH, and catalyst concentration ( outlines factorial methodologies).
- In Situ Monitoring : Employ Raman spectroscopy or inline UV probes to track intermediate formation ( highlights real-time AI-driven adjustments) .
- Byproduct Analysis : Use LC-MS to identify side products (e.g., over-sulfonated species) and adjust stoichiometry (as in ).
Q. Methodological Considerations
Q. What strategies validate the biological or catalytic activity of this compound?
- Enzyme Inhibition Assays : Test interactions with metalloenzymes (e.g., tyrosinase) using kinetic studies (refer to inhibitor analysis in ) .
- Electrochemical Studies : Cyclic voltammetry to assess redox activity linked to Cu²⁺/Cu⁺ transitions ( discusses copper-mediated reactions) .
- Theoretical Alignment : Correlate electronic properties (from DFT) with observed bioactivity ( ’s framework integration) .
Q. How should researchers address discrepancies in reported toxicity or stability profiles?
- Controlled Degradation Studies : Monitor compound stability under UV light, heat, and varying pH (align with ’s safety protocols).
- Comparative Toxicology : Benchmark against structurally similar cuprates ( ) to identify structure-toxicity relationships .
- Ecotoxicology Models : Use Daphnia magna or algal assays to evaluate aquatic toxicity (as per ’s ecological hazard guidelines) .
Q. Tables for Key Data Comparison
Properties
CAS No. |
75199-10-9 |
---|---|
Molecular Formula |
C25H11ClCuFN5Na4O14S4 |
Molecular Weight |
943.6 g/mol |
IUPAC Name |
copper;tetrasodium;7-[(5-chloro-6-fluoro-2-methylpyrimidin-4-yl)amino]-4-oxido-3-[(2-oxido-4,6-disulfonatonaphthalen-1-yl)diazenyl]naphthalene-1,5-disulfonate |
InChI |
InChI=1S/C25H17ClFN5O14S4.Cu.4Na/c1-9-28-24(27)21(26)25(29-9)30-10-4-14-17(48(38,39)40)7-15(23(34)20(14)19(5-10)50(44,45)46)31-32-22-12-3-2-11(47(35,36)37)6-13(12)18(8-16(22)33)49(41,42)43;;;;;/h2-8,33-34H,1H3,(H,28,29,30)(H,35,36,37)(H,38,39,40)(H,41,42,43)(H,44,45,46);;;;;/q;+2;4*+1/p-6 |
InChI Key |
KCTMVHKBTVHYRI-UHFFFAOYSA-H |
Canonical SMILES |
CC1=NC(=C(C(=N1)F)Cl)NC2=CC3=C(C=C(C(=C3C(=C2)S(=O)(=O)[O-])[O-])N=NC4=C5C=CC(=CC5=C(C=C4[O-])S(=O)(=O)[O-])S(=O)(=O)[O-])S(=O)(=O)[O-].[Na+].[Na+].[Na+].[Na+].[Cu+2] |
Origin of Product |
United States |
Disclaimer and Information on In-Vitro Research Products
Please be aware that all articles and product information presented on BenchChem are intended solely for informational purposes. The products available for purchase on BenchChem are specifically designed for in-vitro studies, which are conducted outside of living organisms. In-vitro studies, derived from the Latin term "in glass," involve experiments performed in controlled laboratory settings using cells or tissues. It is important to note that these products are not categorized as medicines or drugs, and they have not received approval from the FDA for the prevention, treatment, or cure of any medical condition, ailment, or disease. We must emphasize that any form of bodily introduction of these products into humans or animals is strictly prohibited by law. It is essential to adhere to these guidelines to ensure compliance with legal and ethical standards in research and experimentation.