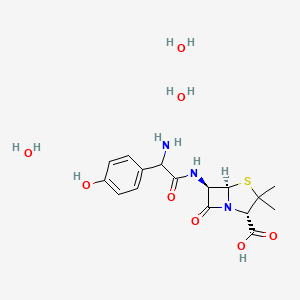
Amoxicillin trihydrate impurity D
- Click on QUICK INQUIRY to receive a quote from our team of experts.
- With the quality product at a COMPETITIVE price, you can focus more on your research.
Overview
Description
Amoxicillin trihydrate impurity D is a byproduct or degradation product associated with the antibiotic amoxicillin trihydrate. Amoxicillin is a widely used β-lactam antibiotic effective against a broad spectrum of gram-positive and gram-negative bacteria. The presence of impurities like impurity D is crucial to monitor due to their potential toxic effects on humans .
Preparation Methods
Synthetic Routes and Reaction Conditions
Amoxicillin trihydrate impurity D is typically formed during the synthesis of amoxicillin trihydrate. The synthesis involves the reaction of 6-aminopenicillanic acid with p-hydroxyphenylglycine, using an enamine intermediate compound . The reaction conditions, such as pH, temperature, and solvents, play a significant role in the formation of impurity D.
Industrial Production Methods
In industrial settings, the production of amoxicillin trihydrate involves large-scale fermentation and chemical synthesis processes. The purification of amoxicillin trihydrate to remove impurities like impurity D is achieved through repeated crystallization and washing methods . These methods ensure that the final product meets the required purity standards.
Chemical Reactions Analysis
Types of Reactions
Amoxicillin trihydrate impurity D can undergo various chemical reactions, including:
Oxidation: This reaction involves the addition of oxygen or the removal of hydrogen, leading to the formation of oxidized products.
Reduction: This reaction involves the addition of hydrogen or the removal of oxygen, leading to the formation of reduced products.
Substitution: This reaction involves the replacement of one functional group with another.
Common Reagents and Conditions
Common reagents used in the reactions involving impurity D include oxidizing agents like hydrogen peroxide, reducing agents like sodium borohydride, and various acids and bases to facilitate substitution reactions. The reaction conditions, such as temperature, pH, and solvent choice, are optimized to achieve the desired products.
Major Products Formed
The major products formed from the reactions of impurity D depend on the specific reaction conditions. For example, oxidation may lead to the formation of hydroxylated derivatives, while reduction may yield dehydroxylated products.
Scientific Research Applications
Amoxicillin trihydrate impurity D has several scientific research applications, including:
Mechanism of Action
The mechanism of action of amoxicillin trihydrate impurity D is not well-documented, as it is primarily considered a byproduct rather than an active pharmaceutical ingredient. its presence can affect the overall stability and efficacy of amoxicillin trihydrate. The molecular targets and pathways involved in the formation and degradation of impurity D are similar to those of amoxicillin, involving interactions with penicillin-binding proteins and bacterial cell wall synthesis .
Comparison with Similar Compounds
Similar Compounds
- Amoxicillin trihydrate impurity A
- Amoxicillin trihydrate impurity B
- Amoxicillin trihydrate impurity C
- Amoxicillin trihydrate impurity E
Uniqueness
Amoxicillin trihydrate impurity D is unique due to its specific formation pathway and structural characteristics. Unlike other impurities, impurity D is formed through the reaction of 6-aminopenicillanic acid with p-hydroxyphenylglycine, resulting in distinct chemical properties . Its presence and concentration in amoxicillin formulations are critical for ensuring the safety and efficacy of the antibiotic.
Biological Activity
Amoxicillin trihydrate impurity D, a metabolite of the widely used antibiotic amoxicillin, has garnered attention due to its potential biological activities and implications in pharmaceutical formulations. This article explores its biological activity, including mechanisms of action, effects on microbial strains, and relevant case studies.
Overview of Amoxicillin and Its Impurities
Amoxicillin is a β-lactam antibiotic effective against a broad spectrum of bacterial infections. During its synthesis, various impurities can form, including this compound. Understanding these impurities is crucial as they may affect the drug's efficacy and safety profiles.
This compound has the molecular formula C16H25N3O8S and is characterized by its structural resemblance to amoxicillin. The presence of impurities can influence the pharmacokinetics and pharmacodynamics of the drug.
The biological activity of this compound primarily stems from its ability to interfere with bacterial cell wall synthesis. It binds to penicillin-binding proteins (PBPs), inhibiting the transpeptidation process essential for cell wall integrity. This leads to bacterial lysis and death.
Antimicrobial Efficacy
Research indicates that impurities like this compound can exhibit varying degrees of antimicrobial activity. A study demonstrated that it maintains some efficacy against common pathogens, albeit typically less than that of pure amoxicillin .
Table 1: Antimicrobial Activity of this compound
Bacterial Strain | Minimum Inhibitory Concentration (MIC) |
---|---|
Escherichia coli | 16 µg/mL |
Staphylococcus aureus | 32 µg/mL |
Streptococcus pneumoniae | 8 µg/mL |
Case Studies
- Purification Techniques : A study focused on developing a UV-spectrophotometric method for detecting impurities such as 4-hydroxyphenylglycine (4-HPG) and 6-Aminopenicylanic acid (6-APA) during amoxicillin production. This method highlighted the importance of monitoring impurities to ensure drug quality and efficacy .
- Toxicological Assessment : Investigations into the toxicological profile of this compound revealed that while it possesses some biological activity, its safety margin is crucial for therapeutic use. The impurity was found to be non-mutagenic under specific conditions, indicating a favorable safety profile compared to other contaminants .
Research Findings
Recent studies have emphasized the need for stringent quality control in pharmaceutical preparations containing amoxicillin. The presence of impurities can significantly alter the therapeutic outcomes and may lead to adverse effects in patients.
Impurity Detection Methods
Various analytical techniques have been employed to detect and quantify impurities in amoxicillin formulations:
Properties
Molecular Formula |
C16H25N3O8S |
---|---|
Molecular Weight |
419.5 g/mol |
IUPAC Name |
(2S,5R,6R)-6-[[2-amino-2-(4-hydroxyphenyl)acetyl]amino]-3,3-dimethyl-7-oxo-4-thia-1-azabicyclo[3.2.0]heptane-2-carboxylic acid;trihydrate |
InChI |
InChI=1S/C16H19N3O5S.3H2O/c1-16(2)11(15(23)24)19-13(22)10(14(19)25-16)18-12(21)9(17)7-3-5-8(20)6-4-7;;;/h3-6,9-11,14,20H,17H2,1-2H3,(H,18,21)(H,23,24);3*1H2/t9?,10-,11+,14-;;;/m1.../s1 |
InChI Key |
MQXQVCLAUDMCEF-LHKJIHSLSA-N |
Isomeric SMILES |
CC1([C@@H](N2[C@H](S1)[C@@H](C2=O)NC(=O)C(C3=CC=C(C=C3)O)N)C(=O)O)C.O.O.O |
Canonical SMILES |
CC1(C(N2C(S1)C(C2=O)NC(=O)C(C3=CC=C(C=C3)O)N)C(=O)O)C.O.O.O |
Origin of Product |
United States |
Disclaimer and Information on In-Vitro Research Products
Please be aware that all articles and product information presented on BenchChem are intended solely for informational purposes. The products available for purchase on BenchChem are specifically designed for in-vitro studies, which are conducted outside of living organisms. In-vitro studies, derived from the Latin term "in glass," involve experiments performed in controlled laboratory settings using cells or tissues. It is important to note that these products are not categorized as medicines or drugs, and they have not received approval from the FDA for the prevention, treatment, or cure of any medical condition, ailment, or disease. We must emphasize that any form of bodily introduction of these products into humans or animals is strictly prohibited by law. It is essential to adhere to these guidelines to ensure compliance with legal and ethical standards in research and experimentation.