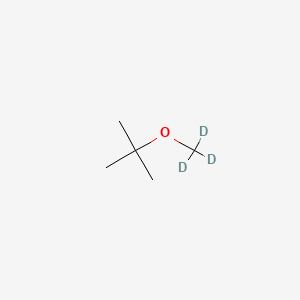
tert-Butyl methyl-d3 ether
Overview
Description
Tert-Butyl methyl-d3 ether is a useful research compound. Its molecular formula is C5H12O and its molecular weight is 91.17 g/mol. The purity is usually 95%.
The exact mass of the compound this compound is unknown and the complexity rating of the compound is unknown. The United Nations designated GHS hazard class pictogram is Flammable;Irritant, and the GHS signal word is DangerThe storage condition is unknown. Please store according to label instructions upon receipt of goods.
BenchChem offers high-quality this compound suitable for many research applications. Different packaging options are available to accommodate customers' requirements. Please inquire for more information about this compound including the price, delivery time, and more detailed information at info@benchchem.com.
Scientific Research Applications
Environmental Impact and Treatment
- Solubility in Aqueous Media : Research on fuel oxygenates like methyl tert-butyl ether (MTBE) and its alternatives has focused on their solubility in water, which is crucial for understanding their environmental fate and transport. Temperature significantly influences their solubility, affecting their potential as groundwater and surface water pollutants (Gonzalez-Olmos & Iglesias, 2008).
- Degradation Pathways : The UV/H2O2 process has been applied to degrade MTBE in aqueous solutions, leading to various primary byproducts and eventual mineralization, showcasing a method to address MTBE contamination (Stefan, Mack, & Bolton, 2000).
Chemical Properties and Reactions
- Vapor-Liquid Equilibria : Studies on the vapor-liquid equilibria of MTBE with various alcohols provide insights into its chemical interactions and properties, which are relevant for its use in the chemical and petrochemical industries (Park, Han, & Gmehling, 2002).
- Esterification and Ritter Reaction : tert-Butyl methyl ether has been effectively used in the esterification of carboxylic acids and in a one-pot esterification and Ritter reaction, demonstrating its versatility in organic synthesis (Dawar, Raju, & Ramakrishna, 2011).
Biodegradation and Metabolism
- Biotransformation by Cytochrome P450 : Human cytochrome P450 2A6 can metabolize MTBE to tert-butyl alcohol, indicating its role in the metabolism of gasoline ethers in the liver and potential human health implications (Shamsipur et al., 2012).
Removal Techniques
- Adsorption Isotherms : The study of adsorption isotherms for MTBE and other fuel oxygenates on activated carbons provides insights into effective methods for their removal from water, important for water treatment technologies (Yu, Adams, & Ludlow, 2005).
- Phytoremediation : The uptake, metabolism, and toxicity of MTBE in weeping willows were studied, showing that plants can remove MTBE from solutions, suggesting phytoremediation as a viable option for contaminated sites (Yu & Gu, 2006).
Sorption Characteristics
- Sorption to Synthetic Resins : Sorption experiments on synthetic resins have shown their affinity for MTBE and TBA, providing data useful for water treatment and passive sampling applications (Bi, Haderlein, & Schmidt, 2005).
Safety and Hazards
Tert-Butyl methyl-d3 ether is a highly flammable liquid and vapor that causes skin irritation . Precautionary measures include keeping away from heat, sparks, open flames, and hot surfaces; using only non-sparking tools; using explosion-proof electrical, ventilating, and lighting equipment; and taking precautionary measures against static discharge .
Mechanism of Action
Target of Action
The primary target of tert-Butyl methyl-d3 ether is the cytochrome P450 enzyme system . This enzyme system plays a crucial role in the metabolism of various substances, including drugs and toxins .
Mode of Action
This compound interacts with its target, the cytochrome P450 enzyme system, through a series of reactions. The ether oxygen of this compound is protonated to form a good leaving group, which can be eliminated as part of an SN2, SN1, or E1 reaction mechanism . The mechanistic pathway is primarily determined by the strong acid used and the type of substituents attached to the ether .
Biochemical Pathways
The metabolism of this compound involves the initial attack by a monooxygenase . In several cases, the enzyme was characterized as a cytochrome P-450 . After oxygenation, the release of a C1-unit as formaldehyde or formate leads to the production of tert-butyl alcohol, which can be converted to 2-hydroxyisobutyric acid and further metabolized .
Pharmacokinetics
This compound is a volatile, flammable, and colorless liquid that is sparingly soluble in water . It has a boiling point of 55-56 °C and a density of 0.765 g/mL at 25 °C . These properties affect the compound’s Absorption, Distribution, Metabolism, and Excretion (ADME) properties, impacting its bioavailability. Absorbed this compound and the resulting metabolites are rapidly exhaled, and to a lesser extent also eliminated via the kidneys .
Result of Action
The molecular and cellular effects of this compound’s action include the transformation of the substance by cytochrome P450 to tert-butyl alcohol and formaldehyde . In humans exposed to high amounts of this compound in drinking water, it might induce programmed cell death .
Action Environment
Environmental factors can influence the action, efficacy, and stability of this compound. For instance, it is very stable under alkaline, neutral, and weakly acidic conditions . In the presence of strong acids, it is cleaved to methanol and isobutene . Furthermore, it is primarily used as a fuel additive, blended into gasoline to increase its octane rating and knock resistance, and reduce unwanted emissions .
Biochemical Analysis
Biochemical Properties
Tert-Butyl methyl-d3 ether plays a significant role in biochemical reactions, especially in lipid extraction. It interacts with various biomolecules, including lipids, proteins, and enzymes. The compound is known for its ability to solubilize small metabolites while precipitating proteins and other macromolecules. This interaction is crucial for the efficient extraction and analysis of lipids from biological samples .
Cellular Effects
This compound influences various cellular processes, including cell signaling pathways, gene expression, and cellular metabolism. Its ability to solubilize lipids and other small metabolites affects the overall cellular function. The compound’s impact on cell signaling pathways and gene expression can lead to changes in cellular metabolism, making it a valuable tool for studying these processes .
Molecular Mechanism
The molecular mechanism of this compound involves its interaction with biomolecules at the molecular level. The compound binds to lipids and other small metabolites, facilitating their extraction and analysis. This binding interaction is essential for the compound’s role in lipidomics and other biochemical studies. Additionally, this compound can inhibit or activate specific enzymes, leading to changes in gene expression and cellular function .
Temporal Effects in Laboratory Settings
In laboratory settings, the effects of this compound can change over time. The compound’s stability and degradation are critical factors that influence its long-term effects on cellular function. Studies have shown that this compound remains stable under specific conditions, allowing for consistent results in in vitro and in vivo experiments. Its degradation over time can affect the accuracy and reliability of experimental outcomes .
Dosage Effects in Animal Models
The effects of this compound vary with different dosages in animal models. At low doses, the compound can effectively solubilize lipids and other small metabolites without causing adverse effects. At high doses, this compound may exhibit toxic or adverse effects, including changes in cellular function and metabolism. Understanding the dosage effects is crucial for optimizing its use in biochemical research .
Metabolic Pathways
This compound is involved in various metabolic pathways, interacting with enzymes and cofactors essential for lipid metabolism. The compound’s ability to solubilize lipids and other small metabolites affects metabolic flux and metabolite levels. This interaction is vital for studying lipid metabolism and other related biochemical processes .
Transport and Distribution
Within cells and tissues, this compound is transported and distributed through specific transporters and binding proteins. These interactions influence the compound’s localization and accumulation, affecting its overall activity and function. Understanding the transport and distribution mechanisms is essential for optimizing the use of this compound in biochemical research .
Subcellular Localization
The subcellular localization of this compound is influenced by targeting signals and post-translational modifications. These factors direct the compound to specific compartments or organelles, affecting its activity and function. Understanding the subcellular localization is crucial for studying the compound’s role in various biochemical processes .
Properties
IUPAC Name |
2-methyl-2-(trideuteriomethoxy)propane | |
---|---|---|
Source | PubChem | |
URL | https://pubchem.ncbi.nlm.nih.gov | |
Description | Data deposited in or computed by PubChem | |
InChI |
InChI=1S/C5H12O/c1-5(2,3)6-4/h1-4H3/i4D3 | |
Source | PubChem | |
URL | https://pubchem.ncbi.nlm.nih.gov | |
Description | Data deposited in or computed by PubChem | |
InChI Key |
BZLVMXJERCGZMT-GKOSEXJESA-N | |
Source | PubChem | |
URL | https://pubchem.ncbi.nlm.nih.gov | |
Description | Data deposited in or computed by PubChem | |
Canonical SMILES |
CC(C)(C)OC | |
Source | PubChem | |
URL | https://pubchem.ncbi.nlm.nih.gov | |
Description | Data deposited in or computed by PubChem | |
Isomeric SMILES |
[2H]C([2H])([2H])OC(C)(C)C | |
Source | PubChem | |
URL | https://pubchem.ncbi.nlm.nih.gov | |
Description | Data deposited in or computed by PubChem | |
Molecular Formula |
C5H12O | |
Source | PubChem | |
URL | https://pubchem.ncbi.nlm.nih.gov | |
Description | Data deposited in or computed by PubChem | |
DSSTOX Substance ID |
DTXSID60583703 | |
Record name | 2-Methyl-2-[(~2~H_3_)methyloxy]propane | |
Source | EPA DSSTox | |
URL | https://comptox.epa.gov/dashboard/DTXSID60583703 | |
Description | DSSTox provides a high quality public chemistry resource for supporting improved predictive toxicology. | |
Molecular Weight |
91.17 g/mol | |
Source | PubChem | |
URL | https://pubchem.ncbi.nlm.nih.gov | |
Description | Data deposited in or computed by PubChem | |
CAS No. |
29366-08-3 | |
Record name | 2-Methyl-2-[(~2~H_3_)methyloxy]propane | |
Source | EPA DSSTox | |
URL | https://comptox.epa.gov/dashboard/DTXSID60583703 | |
Description | DSSTox provides a high quality public chemistry resource for supporting improved predictive toxicology. | |
Record name | tert-Butyl methyl-d3 ether | |
Source | European Chemicals Agency (ECHA) | |
URL | https://echa.europa.eu/information-on-chemicals | |
Description | The European Chemicals Agency (ECHA) is an agency of the European Union which is the driving force among regulatory authorities in implementing the EU's groundbreaking chemicals legislation for the benefit of human health and the environment as well as for innovation and competitiveness. | |
Explanation | Use of the information, documents and data from the ECHA website is subject to the terms and conditions of this Legal Notice, and subject to other binding limitations provided for under applicable law, the information, documents and data made available on the ECHA website may be reproduced, distributed and/or used, totally or in part, for non-commercial purposes provided that ECHA is acknowledged as the source: "Source: European Chemicals Agency, http://echa.europa.eu/". Such acknowledgement must be included in each copy of the material. ECHA permits and encourages organisations and individuals to create links to the ECHA website under the following cumulative conditions: Links can only be made to webpages that provide a link to the Legal Notice page. | |
Disclaimer and Information on In-Vitro Research Products
Please be aware that all articles and product information presented on BenchChem are intended solely for informational purposes. The products available for purchase on BenchChem are specifically designed for in-vitro studies, which are conducted outside of living organisms. In-vitro studies, derived from the Latin term "in glass," involve experiments performed in controlled laboratory settings using cells or tissues. It is important to note that these products are not categorized as medicines or drugs, and they have not received approval from the FDA for the prevention, treatment, or cure of any medical condition, ailment, or disease. We must emphasize that any form of bodily introduction of these products into humans or animals is strictly prohibited by law. It is essential to adhere to these guidelines to ensure compliance with legal and ethical standards in research and experimentation.