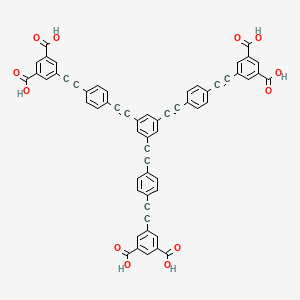
5,5',5''-(((Benzene-1,3,5-triyltris(ethyne-2,1-diyl))tris(benzene-4,1-diyl))tris(ethyne-2,1-diyl))triisophthalic acid
- Click on QUICK INQUIRY to receive a quote from our team of experts.
- With the quality product at a COMPETITIVE price, you can focus more on your research.
Overview
Description
5,5’,5’'-(((Benzene-1,3,5-triyltris(ethyne-2,1-diyl))tris(benzene-4,1-diyl))tris(ethyne-2,1-diyl))triisophthalic acid is a complex organic compound with a molecular formula of C60H30O12 and a molecular weight of 942.87 g/mol This compound is characterized by its intricate structure, which includes multiple benzene rings interconnected by ethyne (acetylene) linkages and isophthalic acid groups
Preparation Methods
The synthesis of 5,5’,5’'-(((Benzene-1,3,5-triyltris(ethyne-2,1-diyl))tris(benzene-4,1-diyl))tris(ethyne-2,1-diyl))triisophthalic acid involves several steps. One common synthetic route includes the following steps :
Starting Materials: The synthesis begins with 1,3,5-tribromobenzene and trimethylsilylacetylene.
Reaction Conditions: The reaction is carried out in an inert atmosphere using a palladium catalyst (Pd(PPh3)2Cl2) and copper iodide (CuI) as a co-catalyst. The reaction mixture is heated to 65°C and stirred for 24 hours.
Intermediate Formation: The intermediate product, 1,3,5-tris((trimethylsilyl)ethynyl)benzene, is obtained.
Deprotection: The trimethylsilyl groups are removed using a fluoride source, such as tetrabutylammonium fluoride (TBAF), to yield the final product.
Industrial production methods may involve similar steps but are optimized for large-scale synthesis, including the use of continuous flow reactors and automated processes to ensure high yield and purity.
Chemical Reactions Analysis
5,5’,5’'-(((Benzene-1,3,5-triyltris(ethyne-2,1-diyl))tris(benzene-4,1-diyl))tris(ethyne-2,1-diyl))triisophthalic acid undergoes various chemical reactions, including:
Oxidation: The compound can undergo oxidation reactions, typically using strong oxidizing agents such as potassium permanganate (KMnO4) or chromium trioxide (CrO3), leading to the formation of carboxylic acids.
Reduction: Reduction reactions can be carried out using reducing agents like lithium aluminum hydride (LiAlH4) or sodium borohydride (NaBH4), resulting in the formation of alcohols.
Substitution: Electrophilic aromatic substitution reactions can occur on the benzene rings, using reagents such as halogens (Cl2, Br2) or nitrating agents (HNO3/H2SO4).
The major products formed from these reactions depend on the specific reagents and conditions used.
Scientific Research Applications
5,5’,5’'-(((Benzene-1,3,5-triyltris(ethyne-2,1-diyl))tris(benzene-4,1-diyl))tris(ethyne-2,1-diyl))triisophthalic acid has several scientific research applications :
Chemistry: It is used as a building block for the synthesis of metal-organic frameworks (MOFs) with ultra-high porosity, which have applications in gas adsorption, separation, and storage.
Biology: The compound’s unique structure allows it to be used in the design of biomimetic materials and drug delivery systems.
Medicine: Research is ongoing to explore its potential use in targeted drug delivery and as a component in diagnostic imaging agents.
Industry: It is used in the development of advanced materials, such as high-performance polymers and nanocomposites.
Mechanism of Action
The mechanism of action of 5,5’,5’'-(((Benzene-1,3,5-triyltris(ethyne-2,1-diyl))tris(benzene-4,1-diyl))tris(ethyne-2,1-diyl))triisophthalic acid involves its ability to form stable complexes with metal ions, which can facilitate various catalytic processes . The spatial separation of triazine and acetylene cores leads to efficient charge separation and suppressed charge recombination, enhancing its performance in catalytic and electronic applications.
Comparison with Similar Compounds
Similar compounds to 5,5’,5’'-(((Benzene-1,3,5-triyltris(ethyne-2,1-diyl))tris(benzene-4,1-diyl))tris(ethyne-2,1-diyl))triisophthalic acid include :
1,3,5-Tris(3,5-dicarboxyphenylethynyl)benzene: This compound has a similar structure but with different functional groups, leading to variations in its chemical properties and applications.
5,5’,5’'-(Benzene-1,3,5-triyltris(ethyne-2,1-diyl))tris(3-(tert-butyl)-2-hydroxybenzaldehyde):
The uniqueness of 5,5’,5’'-(((Benzene-1,3,5-triyltris(ethyne-2,1-diyl))tris(benzene-4,1-diyl))tris(ethyne-2,1-diyl))triisophthalic acid lies in its ability to form highly porous structures and its versatility in various scientific and industrial applications.
Properties
Molecular Formula |
C60H30O12 |
---|---|
Molecular Weight |
942.9 g/mol |
IUPAC Name |
5-[2-[4-[2-[3,5-bis[2-[4-[2-(3,5-dicarboxyphenyl)ethynyl]phenyl]ethynyl]phenyl]ethynyl]phenyl]ethynyl]benzene-1,3-dicarboxylic acid |
InChI |
InChI=1S/C60H30O12/c61-55(62)49-28-46(29-50(34-49)56(63)64)22-16-40-7-1-37(2-8-40)13-19-43-25-44(20-14-38-3-9-41(10-4-38)17-23-47-30-51(57(65)66)35-52(31-47)58(67)68)27-45(26-43)21-15-39-5-11-42(12-6-39)18-24-48-32-53(59(69)70)36-54(33-48)60(71)72/h1-12,25-36H,(H,61,62)(H,63,64)(H,65,66)(H,67,68)(H,69,70)(H,71,72) |
InChI Key |
CPTUKNBVOPDKEL-UHFFFAOYSA-N |
Canonical SMILES |
C1=CC(=CC=C1C#CC2=CC(=CC(=C2)C#CC3=CC=C(C=C3)C#CC4=CC(=CC(=C4)C(=O)O)C(=O)O)C#CC5=CC=C(C=C5)C#CC6=CC(=CC(=C6)C(=O)O)C(=O)O)C#CC7=CC(=CC(=C7)C(=O)O)C(=O)O |
Origin of Product |
United States |
Disclaimer and Information on In-Vitro Research Products
Please be aware that all articles and product information presented on BenchChem are intended solely for informational purposes. The products available for purchase on BenchChem are specifically designed for in-vitro studies, which are conducted outside of living organisms. In-vitro studies, derived from the Latin term "in glass," involve experiments performed in controlled laboratory settings using cells or tissues. It is important to note that these products are not categorized as medicines or drugs, and they have not received approval from the FDA for the prevention, treatment, or cure of any medical condition, ailment, or disease. We must emphasize that any form of bodily introduction of these products into humans or animals is strictly prohibited by law. It is essential to adhere to these guidelines to ensure compliance with legal and ethical standards in research and experimentation.