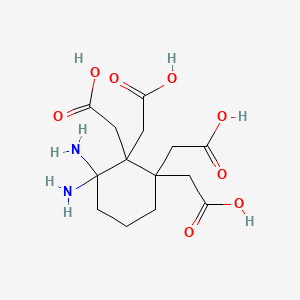
Cyclohexanediaminetetraaceticacid
- Click on QUICK INQUIRY to receive a quote from our team of experts.
- With the quality product at a COMPETITIVE price, you can focus more on your research.
Overview
Description
Cyclohexanediaminetetraacetic acid is a chelating agent that is widely used in various fields due to its ability to form stable complexes with metal ions. It is structurally similar to ethylenediaminetetraacetic acid but contains a cyclohexane ring, which enhances its chelating properties .
Preparation Methods
Cyclohexanediaminetetraacetic acid can be synthesized through several methods. One common method involves the reaction of cyclohexanediamine with chloroacetic acid in the presence of a base. The reaction conditions typically include a temperature range of 60-80°C and a reaction time of 6-8 hours . Industrial production methods often involve the use of more efficient catalysts and purification techniques to achieve higher yields and purity .
Chemical Reactions Analysis
Cyclohexanediaminetetraacetic acid undergoes various chemical reactions, including complexation, oxidation, and reduction. It forms stable complexes with metal ions such as calcium, magnesium, and iron, making it useful in water treatment and analytical chemistry . Common reagents used in these reactions include sodium hydroxide and various metal salts. The major products formed from these reactions are metal-cyclohexanediaminetetraacetic acid complexes .
Scientific Research Applications
Cyclohexanediaminetetraacetic acid has numerous applications in scientific research. In chemistry, it is used as a chelating agent to remove metal ions from solutions and to stabilize metal ions in various reactions . In biology, it is used to study the effects of metal ions on biological systems and to chelate metal ions in enzyme assays . In medicine, it is used in diagnostic imaging and as a potential therapeutic agent for metal ion-related diseases . In industry, it is used in water treatment, textile processing, and as a stabilizer in various chemical processes .
Mechanism of Action
The mechanism of action of cyclohexanediaminetetraacetic acid involves the formation of stable complexes with metal ions. The cyclohexane ring enhances the chelating ability of the compound by providing a rigid structure that can effectively bind metal ions . The molecular targets of cyclohexanediaminetetraacetic acid include various metal ions, and the pathways involved in its action include the formation of metal-ligand complexes and the stabilization of metal ions in solution .
Comparison with Similar Compounds
Cyclohexanediaminetetraacetic acid is similar to other chelating agents such as ethylenediaminetetraacetic acid, nitrilotriacetic acid, and diethylenetriaminepentaacetic acid . it is unique in its ability to form more stable complexes with certain metal ions due to the presence of the cyclohexane ring . This makes it particularly useful in applications where high stability and selectivity are required .
Similar Compounds:- Ethylenediaminetetraacetic acid
- Nitrilotriacetic acid
- Diethylenetriaminepentaacetic acid
Biological Activity
Cyclohexanediaminetetraacetic acid (CDTA) is a synthetic chelating agent that plays a significant role in various biological and environmental applications. This article provides a comprehensive overview of the biological activity of CDTA, focusing on its mechanisms, effects on microbial activity, and therapeutic potential, supported by relevant research findings and case studies.
Overview of CDTA
CDTA, chemically known as trans-1,2-diaminocyclohexane-N,N,N',N'-tetraacetic acid, is a multidentate ligand capable of forming stable complexes with metal ions. Its structure allows it to bind multiple metal ions simultaneously, which is crucial for its applications in biochemistry and medicine.
- Chelation of Metal Ions : CDTA effectively binds to divalent cations such as Ca²⁺ and Mg²⁺, which are essential for microbial cell wall stability. By sequestering these ions, CDTA destabilizes bacterial membranes, leading to increased susceptibility to antimicrobial agents .
- Biofilm Disruption : Studies have shown that CDTA can inhibit biofilm formation by preventing bacterial adhesion and disrupting existing biofilms. This is particularly relevant in clinical settings where biofilms contribute to persistent infections .
- Antimicrobial Activity : CDTA has demonstrated antimicrobial properties against various pathogens. Its ability to enhance the efficacy of other antimicrobial agents makes it a valuable additive in wound care and infection management .
Case Study: Aluminum Chloride Toxicity
A study evaluated the efficacy of CDTA in treating aluminum chloride toxicity in rabbits. The results indicated that CDTA significantly mitigated the toxic effects associated with aluminum exposure, suggesting its potential as a therapeutic agent for heavy metal detoxification .
Table 1: Summary of Biological Activities of CDTA
Detailed Research Findings
- Antimicrobial Efficacy : Research has shown that CDTA can enhance the activity of photodynamic agents against Staphylococcus aureus biofilms by chelating essential ions from the extracellular polymeric substance (EPS) matrix . This mechanism underscores the importance of CDTA in developing novel antimicrobial strategies.
- Crystal Growth Studies : A study on potassium dihydrogen phosphate (KDP) crystals revealed that CDTA affects crystal morphology by reducing step bunching during crystal growth. At optimal concentrations (100 ppm), CDTA improved growth rates by complexing with metal impurities present in the solution .
- In Vivo Studies : The use of CDTA in animal models has provided insights into its therapeutic potential. In cases of heavy metal poisoning, CDTA not only reduced the toxic burden but also improved overall health markers in treated subjects .
Properties
Molecular Formula |
C14H22N2O8 |
---|---|
Molecular Weight |
346.33 g/mol |
IUPAC Name |
2-[3,3-diamino-1,2,2-tris(carboxymethyl)cyclohexyl]acetic acid |
InChI |
InChI=1S/C14H22N2O8/c15-14(16)3-1-2-12(4-8(17)18,5-9(19)20)13(14,6-10(21)22)7-11(23)24/h1-7,15-16H2,(H,17,18)(H,19,20)(H,21,22)(H,23,24) |
InChI Key |
RNMCCPMYXUKHAZ-UHFFFAOYSA-N |
Canonical SMILES |
C1CC(C(C(C1)(N)N)(CC(=O)O)CC(=O)O)(CC(=O)O)CC(=O)O |
Origin of Product |
United States |
Disclaimer and Information on In-Vitro Research Products
Please be aware that all articles and product information presented on BenchChem are intended solely for informational purposes. The products available for purchase on BenchChem are specifically designed for in-vitro studies, which are conducted outside of living organisms. In-vitro studies, derived from the Latin term "in glass," involve experiments performed in controlled laboratory settings using cells or tissues. It is important to note that these products are not categorized as medicines or drugs, and they have not received approval from the FDA for the prevention, treatment, or cure of any medical condition, ailment, or disease. We must emphasize that any form of bodily introduction of these products into humans or animals is strictly prohibited by law. It is essential to adhere to these guidelines to ensure compliance with legal and ethical standards in research and experimentation.