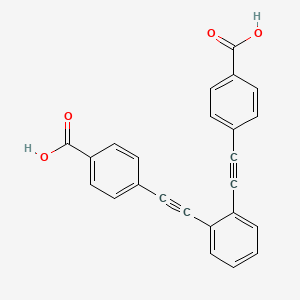
4,4'-(1,2-Phenylenebis(ethyne-2,1-diyl))dibenzoic acid
- Click on QUICK INQUIRY to receive a quote from our team of experts.
- With the quality product at a COMPETITIVE price, you can focus more on your research.
Overview
Description
4,4’-(1,2-Phenylenebis(ethyne-2,1-diyl))dibenzoic acid is a compound with a unique structure characterized by two benzoic acid groups connected via a 1,2-phenylene bridge with ethyne linkages. This compound is known for its rigidity and is often used as a ligand in the formation of metal-organic frameworks (MOFs) due to its ability to form stable structures.
Preparation Methods
Synthetic Routes and Reaction Conditions
The synthesis of 4,4’-(1,2-Phenylenebis(ethyne-2,1-diyl))dibenzoic acid typically involves the coupling of 4-iodobenzoic acid with 1,2-diethynylbenzene under palladium-catalyzed conditions. The reaction is carried out in the presence of a base such as triethylamine and a solvent like dimethylformamide (DMF). The reaction conditions include heating the mixture to a temperature of around 80-100°C for several hours to ensure complete coupling.
Industrial Production Methods
While specific industrial production methods for this compound are not widely documented, the general approach would involve scaling up the laboratory synthesis. This would include optimizing reaction conditions for larger volumes, ensuring efficient mixing and heat transfer, and implementing purification steps such as recrystallization or chromatography to obtain the pure product.
Chemical Reactions Analysis
Types of Reactions
4,4’-(1,2-Phenylenebis(ethyne-2,1-diyl))dibenzoic acid can undergo various chemical reactions, including:
Oxidation: The ethyne linkages can be oxidized to form diketone derivatives.
Reduction: The compound can be reduced to form the corresponding alkane derivatives.
Substitution: The benzoic acid groups can participate in esterification or amidation reactions.
Common Reagents and Conditions
Oxidation: Reagents such as potassium permanganate (KMnO₄) or osmium tetroxide (OsO₄) can be used under acidic or basic conditions.
Reduction: Hydrogenation using palladium on carbon (Pd/C) as a catalyst under hydrogen gas (H₂) atmosphere.
Substitution: Esterification can be carried out using alcohols in the presence of acid catalysts like sulfuric acid (H₂SO₄), while amidation can be achieved using amines and coupling agents like dicyclohexylcarbodiimide (DCC).
Major Products Formed
Oxidation: Formation of diketone derivatives.
Reduction: Formation of alkane derivatives.
Substitution: Formation of esters or amides depending on the reagents used.
Scientific Research Applications
4,4’-(1,2-Phenylenebis(ethyne-2,1-diyl))dibenzoic acid has several scientific research applications:
Chemistry: Used as a ligand in the synthesis of metal-organic frameworks (MOFs) for applications in gas storage, separation, and catalysis.
Biology: Potential use in the development of biosensors due to its ability to form stable complexes with metal ions.
Medicine: Investigated for its potential in drug delivery systems owing to its structural rigidity and ability to form stable complexes.
Industry: Utilized in the development of advanced materials for electronic and photonic applications.
Mechanism of Action
The mechanism of action of 4,4’-(1,2-Phenylenebis(ethyne-2,1-diyl))dibenzoic acid primarily involves its ability to act as a ligand, forming stable complexes with metal ions. These complexes can then participate in various catalytic processes or serve as structural components in MOFs. The ethyne linkages and benzoic acid groups provide sites for interaction with metal ions, facilitating the formation of these complexes.
Comparison with Similar Compounds
Similar Compounds
4,4’-(Anthracene-9,10-diylbis(ethyne-2,1-diyl))dibenzoic acid: Similar structure but with an anthracene core, used in similar applications.
4,4’-(1,4-Phenylenebis(ethyne-2,1-diyl))dibenzoic acid: Slight variation in the position of the ethyne linkages, affecting its reactivity and applications.
Uniqueness
4,4’-(1,2-Phenylenebis(ethyne-2,1-diyl))dibenzoic acid is unique due to its specific structural arrangement, which provides a balance of rigidity and flexibility. This makes it particularly suitable for forming stable MOFs with diverse applications in gas storage, separation, and catalysis.
Properties
Molecular Formula |
C24H14O4 |
---|---|
Molecular Weight |
366.4 g/mol |
IUPAC Name |
4-[2-[2-[2-(4-carboxyphenyl)ethynyl]phenyl]ethynyl]benzoic acid |
InChI |
InChI=1S/C24H14O4/c25-23(26)21-13-7-17(8-14-21)5-11-19-3-1-2-4-20(19)12-6-18-9-15-22(16-10-18)24(27)28/h1-4,7-10,13-16H,(H,25,26)(H,27,28) |
InChI Key |
WGBQCTRKIMJIDQ-UHFFFAOYSA-N |
Canonical SMILES |
C1=CC=C(C(=C1)C#CC2=CC=C(C=C2)C(=O)O)C#CC3=CC=C(C=C3)C(=O)O |
Origin of Product |
United States |
Disclaimer and Information on In-Vitro Research Products
Please be aware that all articles and product information presented on BenchChem are intended solely for informational purposes. The products available for purchase on BenchChem are specifically designed for in-vitro studies, which are conducted outside of living organisms. In-vitro studies, derived from the Latin term "in glass," involve experiments performed in controlled laboratory settings using cells or tissues. It is important to note that these products are not categorized as medicines or drugs, and they have not received approval from the FDA for the prevention, treatment, or cure of any medical condition, ailment, or disease. We must emphasize that any form of bodily introduction of these products into humans or animals is strictly prohibited by law. It is essential to adhere to these guidelines to ensure compliance with legal and ethical standards in research and experimentation.