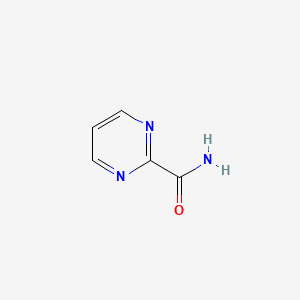
Pyrimidine-2-carboxamide
Overview
Description
Pyrimidine-2-carboxamide is an aromatic heterocyclic compound with the molecular formula C5H5N3O. It contains a pyrimidine ring, which is a six-membered ring with two nitrogen atoms at positions 1 and 3. This compound is known for its diverse pharmacological properties and is used in various scientific research applications .
Scientific Research Applications
Pyrimidine-2-carboxamide has a wide range of applications in scientific research:
Chemistry: It is used as a building block in the synthesis of more complex organic molecules.
Biology: It serves as a precursor for the synthesis of nucleotides and nucleosides, which are essential for DNA and RNA synthesis.
Medicine: It has been investigated for its potential as an anti-inflammatory, antiviral, and anticancer agent.
Industry: It is used in the production of various pharmaceuticals and agrochemicals
Future Directions
Mechanism of Action
Target of Action
It is known that pyrimidine derivatives interact with various enzymes and receptors in the body . For instance, Thieno [2,3-B]Pyridine-2-Carboxamidine, a related compound, has been found to interact with Trypsin-1 and Urokinase-type plasminogen activator in humans .
Mode of Action
These interactions may lead to changes in the conformation or activity of the target, thereby influencing cellular processes .
Biochemical Pathways
2-Pyrimidinecarboxamide likely affects the pyrimidine metabolism pathway. Pyrimidines are essential components of nucleic acids and play a crucial role in many biochemical processes, including DNA and RNA biosynthesis . Disruption of pyrimidine metabolism can have significant effects on cell proliferation and survival .
Pharmacokinetics
Factors such as solubility, permeability, metabolic stability, and elimination rate can influence how much of the compound reaches its target and how long it stays in the body .
Result of Action
This could potentially affect cell growth, differentiation, and survival .
Action Environment
The action, efficacy, and stability of 2-Pyrimidinecarboxamide can be influenced by various environmental factors. These include the pH and temperature of the environment, the presence of other molecules, and the specific characteristics of the target cells. For instance, the efficiency of a reaction involving 2-Pyrimidinecarboxamide may be enhanced under certain conditions, such as in the presence of a catalyst .
Preparation Methods
Synthetic Routes and Reaction Conditions: The synthesis of Pyrimidine-2-carboxamide typically involves the reaction of pyrimidine derivatives with appropriate reagents. One common method is the reaction of 2-chloropyrimidine with ammonia or amines to form the carboxamide derivative. Another approach involves the cyclization of appropriate precursors under specific conditions .
Industrial Production Methods: Industrial production of this compound often employs large-scale chemical reactors where the reaction conditions, such as temperature, pressure, and pH, are carefully controlled to optimize yield and purity. The use of catalysts and solvents may also be involved to enhance the reaction efficiency .
Chemical Reactions Analysis
Types of Reactions: Pyrimidine-2-carboxamide undergoes various chemical reactions, including:
Oxidation: It can be oxidized to form corresponding oxides.
Reduction: Reduction reactions can convert it into amine derivatives.
Substitution: It can undergo nucleophilic substitution reactions where the carboxamide group is replaced by other functional groups.
Common Reagents and Conditions:
Oxidation: Common oxidizing agents include hydrogen peroxide and potassium permanganate.
Reduction: Reducing agents like lithium aluminum hydride (LiAlH4) are often used.
Substitution: Reagents such as alkyl halides and acyl chlorides are used under basic or acidic conditions.
Major Products: The major products formed from these reactions include various substituted pyrimidine derivatives, which can have different pharmacological properties .
Comparison with Similar Compounds
2-Pyridinecarboxamide: Similar in structure but contains a pyridine ring instead of a pyrimidine ring.
2-Pyrazinecarboxamide: Contains a pyrazine ring and is used as an antituberculosis agent.
2-Pyrimidinecarboxamidoxime: A derivative with an oxime group, used in various chemical reactions.
Uniqueness: Pyrimidine-2-carboxamide is unique due to its specific ring structure and the presence of the carboxamide group, which imparts distinct chemical and pharmacological properties. Its ability to participate in a variety of chemical reactions and its wide range of applications in different fields make it a valuable compound in scientific research .
Properties
IUPAC Name |
pyrimidine-2-carboxamide | |
---|---|---|
Source | PubChem | |
URL | https://pubchem.ncbi.nlm.nih.gov | |
Description | Data deposited in or computed by PubChem | |
InChI |
InChI=1S/C5H5N3O/c6-4(9)5-7-2-1-3-8-5/h1-3H,(H2,6,9) | |
Source | PubChem | |
URL | https://pubchem.ncbi.nlm.nih.gov | |
Description | Data deposited in or computed by PubChem | |
InChI Key |
FUXJMHXHGDAHPD-UHFFFAOYSA-N | |
Source | PubChem | |
URL | https://pubchem.ncbi.nlm.nih.gov | |
Description | Data deposited in or computed by PubChem | |
Canonical SMILES |
C1=CN=C(N=C1)C(=O)N | |
Source | PubChem | |
URL | https://pubchem.ncbi.nlm.nih.gov | |
Description | Data deposited in or computed by PubChem | |
Molecular Formula |
C5H5N3O | |
Source | PubChem | |
URL | https://pubchem.ncbi.nlm.nih.gov | |
Description | Data deposited in or computed by PubChem | |
DSSTOX Substance ID |
DTXSID50878771 | |
Record name | 2-PYRIMIDINECARBOXAMIDE | |
Source | EPA DSSTox | |
URL | https://comptox.epa.gov/dashboard/DTXSID50878771 | |
Description | DSSTox provides a high quality public chemistry resource for supporting improved predictive toxicology. | |
Molecular Weight |
123.11 g/mol | |
Source | PubChem | |
URL | https://pubchem.ncbi.nlm.nih.gov | |
Description | Data deposited in or computed by PubChem | |
CAS No. |
88511-48-2 | |
Record name | 2-PYRIMIDINECARBOXAMIDE | |
Source | EPA DSSTox | |
URL | https://comptox.epa.gov/dashboard/DTXSID50878771 | |
Description | DSSTox provides a high quality public chemistry resource for supporting improved predictive toxicology. | |
Synthesis routes and methods
Procedure details
Retrosynthesis Analysis
AI-Powered Synthesis Planning: Our tool employs the Template_relevance Pistachio, Template_relevance Bkms_metabolic, Template_relevance Pistachio_ringbreaker, Template_relevance Reaxys, Template_relevance Reaxys_biocatalysis model, leveraging a vast database of chemical reactions to predict feasible synthetic routes.
One-Step Synthesis Focus: Specifically designed for one-step synthesis, it provides concise and direct routes for your target compounds, streamlining the synthesis process.
Accurate Predictions: Utilizing the extensive PISTACHIO, BKMS_METABOLIC, PISTACHIO_RINGBREAKER, REAXYS, REAXYS_BIOCATALYSIS database, our tool offers high-accuracy predictions, reflecting the latest in chemical research and data.
Strategy Settings
Precursor scoring | Relevance Heuristic |
---|---|
Min. plausibility | 0.01 |
Model | Template_relevance |
Template Set | Pistachio/Bkms_metabolic/Pistachio_ringbreaker/Reaxys/Reaxys_biocatalysis |
Top-N result to add to graph | 6 |
Feasible Synthetic Routes
Q1: What is the significance of studying the coordination chemistry of 2-pyrimidinecarboxamide with metal ions like silver and cadmium?
A1: Investigating the interaction of 2-pyrimidinecarboxamide with metal ions is crucial for several reasons. Firstly, it provides insights into the coordination behavior of this ligand, revealing its potential to form complexes with different metal centers. This understanding is essential for designing new materials with tailored properties. For instance, the study ["Ribbon structure of the coordination polymer of silver trifluoroacetate with 2-pyrimidinecarboxamide"] [] investigated the formation of a coordination polymer, demonstrating the ability of 2-pyrimidinecarboxamide to act as a bridging ligand. This has implications for creating materials with unique structural arrangements and potential applications in areas like catalysis and sensing. Similarly, the research on its interaction with cadmium [] broadens the understanding of its coordination capabilities with various metals.
Q2: Can you elaborate on the structural features of 2-pyrimidinecarboxamide and its coordination with silver as reported in the research?
A2: While the provided abstract doesn't offer detailed spectroscopic data for 2-pyrimidinecarboxamide, it does mention a key structural characteristic of its complex with silver trifluoroacetate: a ribbon-like structure []. This suggests that 2-pyrimidinecarboxamide acts as a bridging ligand, connecting silver ions to form a polymeric chain. The specific arrangement and bonding interactions within this ribbon structure would require further investigation using techniques like X-ray crystallography. Understanding these structural details is essential for correlating the compound's structure with its properties and potential applications.
Disclaimer and Information on In-Vitro Research Products
Please be aware that all articles and product information presented on BenchChem are intended solely for informational purposes. The products available for purchase on BenchChem are specifically designed for in-vitro studies, which are conducted outside of living organisms. In-vitro studies, derived from the Latin term "in glass," involve experiments performed in controlled laboratory settings using cells or tissues. It is important to note that these products are not categorized as medicines or drugs, and they have not received approval from the FDA for the prevention, treatment, or cure of any medical condition, ailment, or disease. We must emphasize that any form of bodily introduction of these products into humans or animals is strictly prohibited by law. It is essential to adhere to these guidelines to ensure compliance with legal and ethical standards in research and experimentation.