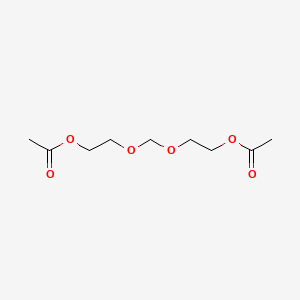
(Methylenebis(oxy))bis(ethane-2,1-diyl) diacetate
- Click on QUICK INQUIRY to receive a quote from our team of experts.
- With the quality product at a COMPETITIVE price, you can focus more on your research.
Overview
Description
(Methylenebis(oxy))bis(ethane-2,1-diyl) diacetate is an organic compound with the molecular formula C9H16O6. It is a diester derived from ethylene glycol and acetic acid. This compound is known for its applications in various fields, including chemistry, biology, and industry.
Preparation Methods
Synthetic Routes and Reaction Conditions
The synthesis of (Methylenebis(oxy))bis(ethane-2,1-diyl) diacetate typically involves the esterification of ethylene glycol with acetic acid. The reaction is catalyzed by an acid catalyst, such as sulfuric acid, and is carried out under reflux conditions. The reaction can be represented as follows:
HOCH2CH2OH+2CH3COOH→CH3COOCH2CH2OCOCH3+2H2O
Industrial Production Methods
In industrial settings, the production of this compound involves continuous esterification processes. The reaction is carried out in large reactors with efficient mixing and temperature control to ensure high yield and purity. The use of azeotropic distillation helps in the removal of water formed during the reaction, driving the equilibrium towards the formation of the ester.
Chemical Reactions Analysis
Types of Reactions
(Methylenebis(oxy))bis(ethane-2,1-diyl) diacetate undergoes various chemical reactions, including:
Hydrolysis: The ester bonds can be hydrolyzed in the presence of water and an acid or base catalyst, yielding ethylene glycol and acetic acid.
Transesterification: The compound can react with other alcohols in the presence of a catalyst to form different esters.
Oxidation: Under strong oxidative conditions, the compound can be oxidized to form corresponding carboxylic acids.
Common Reagents and Conditions
Hydrolysis: Acidic or basic conditions with water.
Transesterification: Alcohols and a catalyst such as sodium methoxide.
Oxidation: Strong oxidizing agents like potassium permanganate or chromic acid.
Major Products
Hydrolysis: Ethylene glycol and acetic acid.
Transesterification: Different esters depending on the alcohol used.
Oxidation: Corresponding carboxylic acids.
Scientific Research Applications
(Methylenebis(oxy))bis(ethane-2,1-diyl) diacetate has several applications in scientific research:
Chemistry: Used as a reagent in organic synthesis and as a solvent in various chemical reactions.
Biology: Employed in the preparation of biological samples and as a component in certain biochemical assays.
Medicine: Investigated for its potential use in drug delivery systems due to its biocompatibility and ability to form stable complexes with various drugs.
Industry: Utilized in the production of polymers, resins, and plasticizers.
Mechanism of Action
The mechanism of action of (Methylenebis(oxy))bis(ethane-2,1-diyl) diacetate involves its ability to form ester bonds with various substrates. In biological systems, it can interact with cellular components through esterification and transesterification reactions. The molecular targets and pathways involved include enzymes that catalyze ester bond formation and hydrolysis.
Comparison with Similar Compounds
Similar Compounds
- Diethylene glycol diacetate
- Triethylene glycol diacetate
- Ethylene glycol diacetate
Comparison
(Methylenebis(oxy))bis(ethane-2,1-diyl) diacetate is unique due to its specific molecular structure, which provides distinct physical and chemical properties. Compared to similar compounds, it has a balanced hydrophilic-lipophilic nature, making it suitable for various applications in both aqueous and organic environments.
Properties
Molecular Formula |
C9H16O6 |
---|---|
Molecular Weight |
220.22 g/mol |
IUPAC Name |
2-(2-acetyloxyethoxymethoxy)ethyl acetate |
InChI |
InChI=1S/C9H16O6/c1-8(10)14-5-3-12-7-13-4-6-15-9(2)11/h3-7H2,1-2H3 |
InChI Key |
ZHAHEJYDNRZNGZ-UHFFFAOYSA-N |
Canonical SMILES |
CC(=O)OCCOCOCCOC(=O)C |
Origin of Product |
United States |
Disclaimer and Information on In-Vitro Research Products
Please be aware that all articles and product information presented on BenchChem are intended solely for informational purposes. The products available for purchase on BenchChem are specifically designed for in-vitro studies, which are conducted outside of living organisms. In-vitro studies, derived from the Latin term "in glass," involve experiments performed in controlled laboratory settings using cells or tissues. It is important to note that these products are not categorized as medicines or drugs, and they have not received approval from the FDA for the prevention, treatment, or cure of any medical condition, ailment, or disease. We must emphasize that any form of bodily introduction of these products into humans or animals is strictly prohibited by law. It is essential to adhere to these guidelines to ensure compliance with legal and ethical standards in research and experimentation.