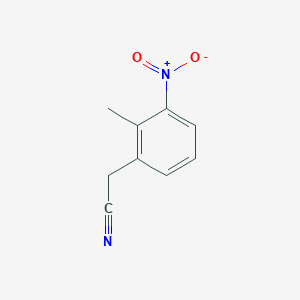
2-Methyl-3-nitrobenzyl cyanide
Overview
Description
2-Methyl-3-nitrobenzyl cyanide (C₉H₈N₂O₂, molecular weight: 176.18 g/mol) is a nitroaromatic cyanide derivative synthesized via a multi-step process starting from o-xylene. The synthesis involves nitration, oxidation, hydrolysis, reduction, chlorination, and cyanation . This compound serves as a critical intermediate in the production of dopamine D2 receptor agonists, such as ropinirole, used in Parkinson’s disease therapy .
Preparation Methods
Direct Nitration of 2-Methylphenylacetic Acid
This method bypasses complex multi-step synthesis by employing 2-methylphenylacetic acid as a precursor. The reaction utilizes a nitric acid–acetic anhydride system in dichloromethane, achieving selective nitration at the 3-position of the aromatic ring.
Reaction Conditions
Parameter | Value |
---|---|
Molar Ratio (Substrate:HNO₃:Ac₂O) | 1:1.5:1.1 |
Temperature | 0–5°C |
Reaction Time | 1–3 hours |
Solvent | Dichloromethane |
Procedure :
- Nitration : 2-Methylphenylacetic acid, acetic anhydride, and dichloromethane are mixed in a flask cooled to 0°C. Concentrated nitric acid (98%) is added dropwise.
- Post-Reaction : The mixture is maintained at 0–5°C for 1–3 hours, then filtered to isolate the crude product.
Yield : 59.6–61.4% (varies with nitric acid stoichiometry).
Purity : Confirmed via IR (NO₂ peaks at 1526 cm⁻¹, 1340 cm⁻¹), ¹H-NMR (δ 12.56 ppm for COOH proton), and elemental analysis (C: 55.38–55.42%, N: 7.14–7.23%).
Advantages :
- Single-step synthesis reduces cumulative error.
- Low-cost starting material (2-methylphenylacetic acid is commercially available).
Limitations :
- Moderate yield due to competing side reactions.
- Requires strict temperature control to prevent over-nitration.
Multi-Step Synthesis from o-Xylene
This route involves sequential functional group transformations, starting with nitration, followed by oxidation, hydrolysis, reduction, chlorination, and cyanation.
Stepwise Reaction Pathway
- Nitration : o-Xylene → 2-Methyl-3-nitrotoluene.
- Oxidation : Methyl group oxidized to carboxylic acid (2-methyl-3-nitrobenzoic acid).
- Reduction : Nitro group reduced to amine (2-methyl-3-aminobenzoic acid).
- Chlorination : Amine converted to chloro derivative (2-methyl-3-chlorobenzoic acid).
- Cyanation : Chloride displaced by cyanide (2-methyl-3-nitrobenzyl cyanide).
Key Reagents :
- Nitration : HNO₃/H₂SO₄.
- Oxidation : KMnO₄ or CrO₃.
- Reduction : H₂/Pd-C or Fe/HCl.
- Cyanation : KCN or NaCN.
Yield : ~40–50% (cumulative yield across steps).
Purity :
- IR : C≡N stretch at 2240 cm⁻¹, NO₂ peaks at 1520 cm⁻¹.
- ¹H-NMR : δ 3.79 ppm (CH₂CN), δ 2.28 ppm (CH₃).
Advantages :
- Flexibility in intermediate purification.
- Well-established protocols for each step.
Limitations :
- Low overall yield due to multiple steps.
- Hazardous reagents (e.g., HNO₃, CrO₃).
Industrial-Scale Optimizations
Industrial methods prioritize cost, safety, and scalability:
Optimization Strategy | Implementation |
---|---|
Catalyst Use | Pd/C or Cu catalysts for reduction steps. |
Solvent Recovery | Dichloromethane recycled via distillation. |
Continuous Flow | Microreactors for nitration to improve heat dissipation. |
Waste Management | Cyanide byproducts treated with NaOH/H₂O₂. |
Case Study :
A process using this compound as a pharmaceutical intermediate achieved 92% purity via crystallization in methanol.
Analytical Characterization Data
Technique | Data (this compound) |
---|---|
IR (cm⁻¹) | 2240 (C≡N), 1718 (COOH), 1526/1340 (NO₂) |
¹H-NMR (δ, ppm) | 12.56 (COOH), 7.37–7.74 (aromatic), 3.79 (CH₂CN) |
Mass Spectrometry | m/z 195 ([M]⁺) |
Melting Point | 72–73°C (crystallized from methanol) |
Critical Challenges and Solutions
Chemical Reactions Analysis
Types of Reactions
2-Methyl-3-nitrobenzyl cyanide undergoes various types of chemical reactions, including:
Reduction: The nitro group can be reduced to an amino group using reducing agents such as hydrogen gas in the presence of a catalyst (e.g., palladium on carbon).
Substitution: The cyanide group can participate in nucleophilic substitution reactions, where it is replaced by other nucleophiles.
Oxidation: The methyl group can be oxidized to form a carboxylic acid group.
Common Reagents and Conditions
Reduction: Hydrogen gas with palladium on carbon as a catalyst.
Substitution: Nucleophiles such as amines or alcohols under basic conditions.
Oxidation: Strong oxidizing agents such as potassium permanganate or chromium trioxide.
Major Products
Reduction: 2-Methyl-3-aminobenzyl cyanide.
Substitution: Various substituted benzyl cyanides depending on the nucleophile used.
Oxidation: 2-Methyl-3-nitrobenzoic acid.
Scientific Research Applications
Organic Synthesis
2-Methyl-3-nitrobenzyl cyanide serves as an important intermediate in the synthesis of more complex organic molecules. Its ability to undergo various chemical reactions, such as reduction and substitution, makes it a versatile building block for creating diverse compounds .
Pharmaceuticals
The compound is particularly notable for its potential applications in drug development. Research indicates that this compound can be utilized in synthesizing pharmaceuticals aimed at treating conditions like Parkinson's disease and restless legs syndrome . Its structure allows for modifications that enhance biological activity, making it a candidate for antimicrobial and anticancer agents.
Material Science
In material science, this compound is employed in the synthesis of polymers and specialty chemicals. Its unique chemical properties allow for the development of materials with specific functionalities suitable for various industrial applications.
Chemical Industry
The compound is also utilized as a precursor in the production of dyes, pigments, and other specialty chemicals. Its reactivity facilitates the creation of colorants and functional materials that are essential in various industries .
Data Table: Chemical Reactions Involving this compound
Reaction Type | Description | Major Products |
---|---|---|
Reduction | Reduction of nitro group to amino group using hydrogen gas | 2-Methyl-3-aminobenzyl cyanide |
Substitution | Nucleophilic substitution reactions involving the cyanide group | Various substituted benzyl cyanides |
Oxidation | Oxidation of methyl group to form carboxylic acid | 2-Methyl-3-nitrobenzoic acid |
Case Study 1: Pharmaceutical Development
A study focusing on the synthesis of derivatives of this compound highlighted its potential in developing new treatments for neurodegenerative diseases. The modifications made to its structure resulted in compounds exhibiting enhanced efficacy against target pathways involved in Parkinson's disease .
Case Study 2: Material Science Innovations
Research conducted on the use of this compound in polymer synthesis demonstrated its ability to improve mechanical properties and thermal stability of produced materials. The findings suggest that incorporating this compound into polymer matrices can lead to innovative applications in coatings and composites .
Mechanism of Action
The mechanism of action of 2-Methyl-3-nitrobenzyl cyanide depends on the specific chemical reactions it undergoes. For example, in reduction reactions, the nitro group is reduced to an amino group through the transfer of electrons and protons. In substitution reactions, the cyanide group is replaced by a nucleophile through a nucleophilic attack on the carbon atom.
Comparison with Similar Compounds
Structural and Crystallographic Properties
The compound crystallizes in a monoclinic system (space group P2₁/c) with unit cell parameters:
- a = 17.216 Å, b = 7.1950 Å, c = 15.746 Å, β = 117.10°, V = 1736.3 ų, Z = 8 . The asymmetric unit contains two molecules with a dihedral angle of 20.15° between aromatic rings, stabilized by C–H···O hydrogen bonds . The nitro (NO₂) and cyanide (CN) groups contribute to its planar geometry and electronic properties, as confirmed by X-ray diffraction studies using SHELX software .
Comparison with Structurally Related Compounds
3-Nitrobenzyl Cyanide (Without Methyl Group)
- Molecular Formula : C₈H₆N₂O₂.
- Key Differences : The absence of the methyl group at the 2-position reduces steric hindrance and lipophilicity. This may alter solubility and reactivity in substitution reactions.
- Reactivity : The nitro group’s electron-withdrawing effect enhances electrophilic substitution at the para position. The methyl group in 2-methyl-3-nitrobenzyl cyanide likely directs reactions to specific sites, influencing its utility in pharmaceutical synthesis .
2-Methylbenzyl Cyanide (Without Nitro Group)
- Molecular Formula : C₉H₉N.
- Key Differences : The lack of a nitro group diminishes electron-withdrawing effects, reducing stability toward nucleophilic attack. This analog is less reactive in nitration or oxidation steps, limiting its use in complex syntheses .
N-(2-Hydroxy-1,1-dimethylethyl)-3-methylbenzamide
- Molecular Features : Contains a benzamide core with a hydroxyl and dimethyl group.
- In contrast, this compound’s nitro and cyanide groups may act as dual directing groups, enabling regioselective modifications in drug synthesis .
Physicochemical and Functional Comparisons
Physical Properties
Property | This compound | 3-Nitrobenzyl Cyanide |
---|---|---|
Molecular Weight | 176.18 g/mol | 162.15 g/mol |
Crystal System | Monoclinic (P2₁/c) | Likely similar |
Melting Point | Not reported | ~100–110°C (estimated) |
Solubility | Moderate in polar solvents | Higher in polar solvents due to reduced steric effects |
Biological Activity
2-Methyl-3-nitrobenzyl cyanide (C9H8N2O2) is a compound that has garnered attention due to its potential biological activities and applications in medicinal chemistry. This article explores its biological activity, mechanisms of action, and relevant research findings.
This compound can be synthesized through a series of chemical reactions, starting from o-xylene. The synthesis involves nitration, oxidation, hydrolysis, reduction, chlorination, and cyanation processes . The molecular structure includes a nitro group that significantly influences its reactivity and biological interactions.
The biological activity of this compound is primarily attributed to its ability to interact with various biomolecules. Key mechanisms include:
- Enzyme Interaction : The compound can act as an enzyme inhibitor or activator, modulating enzymatic pathways that are crucial for cellular functions. Its nitro group is particularly reactive, participating in redox reactions that alter the oxidation states of biomolecules .
- Transport and Distribution : Within biological systems, the compound's transport is facilitated by specific transporters and binding proteins. Its localization in tissues can affect its efficacy.
- Subcellular Localization : The compound can target specific organelles within cells, enhancing its biological effects by ensuring that it acts in the appropriate cellular context.
Antiparkinsonian Effects
Research indicates that this compound may have therapeutic potential for Parkinson's disease (PD) and restless legs syndrome (RLS). The compound has been noted for its ability to influence dopaminergic pathways, which are critical in the treatment of PD .
Toxicity and Dosage Effects
Studies show that the effects of this compound vary with dosage:
- Low Doses : At lower concentrations, the compound may enhance metabolic activity or promote beneficial gene expression.
- High Doses : Conversely, higher doses can lead to toxicity, causing cellular damage and disrupting normal metabolic processes. Threshold effects indicate a specific dosage range where the compound is effective without adverse effects.
Case Studies
Several studies have investigated the biological activity of this compound:
- Therapeutic Applications : In one study, the compound was evaluated for its efficacy in animal models of PD. Results indicated significant improvements in motor functions compared to control groups .
- Toxicological Assessments : Another research effort focused on understanding the toxicological profile of the compound. It was found that prolonged exposure at high concentrations led to oxidative stress markers in liver tissues of treated animals.
Summary of Biological Activities
Q & A
Basic Research Questions
Q. What are the established synthetic routes for 2-methyl-3-nitrobenzyl cyanide, and what experimental conditions optimize yield?
The compound is synthesized via a multi-step route starting from o-xylene. Key steps include nitration, oxidation, hydrolysis, reduction, chlorination, and cyanation. For crystallization, dissolving 2.0 mmol of the compound in methanol (25 mL) and slow evaporation at 298 K over four days yields block-shaped yellow crystals suitable for X-ray diffraction . Optimal reaction conditions (e.g., temperature, solvent ratios) should be validated using thin-layer chromatography (TLC) and nuclear magnetic resonance (NMR) spectroscopy to monitor intermediate purity.
Q. How is single-crystal X-ray diffraction (SC-XRD) applied to determine the molecular structure of this compound?
SC-XRD analysis reveals a monoclinic crystal system (space group P2₁/c) with unit cell parameters a = 17.216 Å, b = 7.195 Å, c = 15.746 Å, and β = 117.10°. The asymmetric unit contains two molecules with a dihedral angle of 20.15° between aromatic rings. Data collection using an Enraf–Nonius CAD-4 diffractometer (MoKα radiation, λ = 0.71073 Å) and refinement via SHELXL97 software provide a final R factor of 0.073 . Researchers should ensure absorption correction (ψ-scan) and validate data quality using Rint (<0.029) .
Q. What are the critical crystallographic parameters for characterizing this compound?
Key parameters include:
- Space group: P2₁/c
- Unit cell volume: 1736.3 ų
- Z = 8 (molecules per unit cell)
- Density: 1.348 Mg/m³ These metrics are essential for comparative studies of polymorphism or co-crystallization experiments .
Advanced Research Questions
Q. How can researchers address high R-factor discrepancies during crystallographic refinement?
The reported wR(F²) of 0.197 suggests challenges in modeling disordered regions or hydrogen bonding. To mitigate this:
- Apply anisotropic displacement parameters for non-hydrogen atoms.
- Constrain H-atom positions using riding models (C–H = 0.93–0.97 Å) and refine isotropically (Uiso = 1.2–1.5 × Ueq of carrier atoms) .
- Cross-validate with Hirshfeld surface analysis to assess intermolecular interactions.
Q. What methodological strategies resolve contradictions between spectroscopic and crystallographic data?
Discrepancies in nitro or cyanide group orientations can arise from dynamic effects in solution (NMR) versus static crystal packing (SC-XRD). To reconcile:
- Perform variable-temperature NMR to probe conformational flexibility.
- Use density functional theory (DFT) calculations to compare optimized gas-phase structures with XRD-derived geometries .
- Employ Raman spectroscopy to validate vibrational modes of nitro and cyanide groups .
Q. How do hydrogen-bonding interactions influence the crystal packing of this compound?
The crystal lattice exhibits C–H···O hydrogen bonds (Table 2 in ), with donor-acceptor distances of 2.5–3.0 Å. These interactions stabilize the asymmetric unit’s 20.15° dihedral angle. Researchers should map interaction networks using software like Mercury (CCDC) and quantify their contribution to lattice energy via Pixel electrostatic potential calculations .
Q. What advanced techniques validate the compound’s purity and stability under experimental conditions?
- High-resolution mass spectrometry (HRMS): Confirm molecular ion peaks (m/z = 176.18 for [M+H]⁺) and rule out degradation products.
- Thermogravimetric analysis (TGA): Assess thermal stability up to decomposition temperatures (~250°C).
- HPLC-DAD: Monitor batch-to-batch consistency using a C18 column (acetonitrile/water mobile phase) .
Q. Methodological Considerations
- Data Quality Control: For SC-XRD, ensure I > 2σ(I) criteria and apply multi-scan absorption corrections (Tmin/max = 0.962/0.981) to minimize systematic errors .
- Software Tools: Use SHELXTL for structure visualization and PLATON for symmetry validation .
- Safety Protocols: Adhere to cyanide management guidelines (e.g., Cyanide Code ) during synthesis and waste disposal.
Properties
IUPAC Name |
2-(2-methyl-3-nitrophenyl)acetonitrile | |
---|---|---|
Source | PubChem | |
URL | https://pubchem.ncbi.nlm.nih.gov | |
Description | Data deposited in or computed by PubChem | |
InChI |
InChI=1S/C9H8N2O2/c1-7-8(5-6-10)3-2-4-9(7)11(12)13/h2-4H,5H2,1H3 | |
Source | PubChem | |
URL | https://pubchem.ncbi.nlm.nih.gov | |
Description | Data deposited in or computed by PubChem | |
InChI Key |
CFNZYZHZLNOYFQ-UHFFFAOYSA-N | |
Source | PubChem | |
URL | https://pubchem.ncbi.nlm.nih.gov | |
Description | Data deposited in or computed by PubChem | |
Canonical SMILES |
CC1=C(C=CC=C1[N+](=O)[O-])CC#N | |
Source | PubChem | |
URL | https://pubchem.ncbi.nlm.nih.gov | |
Description | Data deposited in or computed by PubChem | |
Molecular Formula |
C9H8N2O2 | |
Source | PubChem | |
URL | https://pubchem.ncbi.nlm.nih.gov | |
Description | Data deposited in or computed by PubChem | |
DSSTOX Substance ID |
DTXSID80543081 | |
Record name | (2-Methyl-3-nitrophenyl)acetonitrile | |
Source | EPA DSSTox | |
URL | https://comptox.epa.gov/dashboard/DTXSID80543081 | |
Description | DSSTox provides a high quality public chemistry resource for supporting improved predictive toxicology. | |
Molecular Weight |
176.17 g/mol | |
Source | PubChem | |
URL | https://pubchem.ncbi.nlm.nih.gov | |
Description | Data deposited in or computed by PubChem | |
CAS No. |
23876-14-4 | |
Record name | (2-Methyl-3-nitrophenyl)acetonitrile | |
Source | EPA DSSTox | |
URL | https://comptox.epa.gov/dashboard/DTXSID80543081 | |
Description | DSSTox provides a high quality public chemistry resource for supporting improved predictive toxicology. | |
Synthesis routes and methods I
Procedure details
Synthesis routes and methods II
Procedure details
Synthesis routes and methods III
Procedure details
Retrosynthesis Analysis
AI-Powered Synthesis Planning: Our tool employs the Template_relevance Pistachio, Template_relevance Bkms_metabolic, Template_relevance Pistachio_ringbreaker, Template_relevance Reaxys, Template_relevance Reaxys_biocatalysis model, leveraging a vast database of chemical reactions to predict feasible synthetic routes.
One-Step Synthesis Focus: Specifically designed for one-step synthesis, it provides concise and direct routes for your target compounds, streamlining the synthesis process.
Accurate Predictions: Utilizing the extensive PISTACHIO, BKMS_METABOLIC, PISTACHIO_RINGBREAKER, REAXYS, REAXYS_BIOCATALYSIS database, our tool offers high-accuracy predictions, reflecting the latest in chemical research and data.
Strategy Settings
Precursor scoring | Relevance Heuristic |
---|---|
Min. plausibility | 0.01 |
Model | Template_relevance |
Template Set | Pistachio/Bkms_metabolic/Pistachio_ringbreaker/Reaxys/Reaxys_biocatalysis |
Top-N result to add to graph | 6 |
Feasible Synthetic Routes
Disclaimer and Information on In-Vitro Research Products
Please be aware that all articles and product information presented on BenchChem are intended solely for informational purposes. The products available for purchase on BenchChem are specifically designed for in-vitro studies, which are conducted outside of living organisms. In-vitro studies, derived from the Latin term "in glass," involve experiments performed in controlled laboratory settings using cells or tissues. It is important to note that these products are not categorized as medicines or drugs, and they have not received approval from the FDA for the prevention, treatment, or cure of any medical condition, ailment, or disease. We must emphasize that any form of bodily introduction of these products into humans or animals is strictly prohibited by law. It is essential to adhere to these guidelines to ensure compliance with legal and ethical standards in research and experimentation.