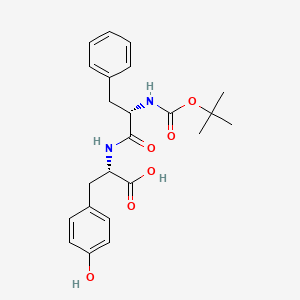
Boc-Phe-Tyr-OH
- Click on QUICK INQUIRY to receive a quote from our team of experts.
- With the quality product at a COMPETITIVE price, you can focus more on your research.
Overview
Description
Boc-Phe-Tyr-OH is a complex organic compound with significant importance in various fields of scientific research. This compound is characterized by its intricate structure, which includes a hydroxyphenyl group, a phenylpropanoyl group, and a tert-butoxycarbonyl group. Its unique configuration and functional groups make it a subject of interest in synthetic chemistry, pharmacology, and material science.
Mechanism of Action
Target of Action
The primary targets of (2S)-3-(4-Hydroxyphenyl)-2-[[(2S)-2-[(2-methylpropan-2-yl)oxycarbonylamino]-3-phenylpropanoyl]amino]propanoic acid are transporter-related nutrient sensors, also known as transceptors . These transceptors mediate nutrient activation of signaling pathways through the plasma membrane .
Mode of Action
The compound interacts with its targets, the transceptors, to mediate nutrient activation of signaling pathways . The exact mechanism of action of transporting and non-transporting transceptors is still unknown .
Biochemical Pathways
The compound, also known as Boc-Phe-Tyr-OH, can be introduced into the peptide structure and used as photoswitchable units for peptide modifications . It is prepared from protected tyrosine in the Mannich reaction, and further used as a building block in peptide synthesis . Moreover, peptides containing tyrosine can be transformed into a photoactivable quinone methide (QM) precursor by the Mannich reaction .
Pharmacokinetics
It’s worth noting that the compound is used in peptide synthesis, which suggests that its absorption, distribution, metabolism, and excretion (adme) properties would be influenced by the peptide it is incorporated into .
Result of Action
The compound’s action results in the formation of quinone methides (QMs) upon electronic excitation, leading to the reactions of peptides with different reagents . This ability to photochemically trigger peptide modifications and interactions with other molecules can have numerous applications in organic synthesis, materials science, biology, and medicine .
Action Environment
The action, efficacy, and stability of (2S)-3-(4-Hydroxyphenyl)-2-[[(2S)-2-[(2-methylpropan-2-yl)oxycarbonylamino]-3-phenylpropanoyl]amino]propanoic acid can be influenced by various environmental factors. For instance, the compound’s photochemical reactivity can be studied by preparative irradiation in methanol, where photodeamination and photomethanolysis occur .
Preparation Methods
Synthetic Routes and Reaction Conditions
The synthesis of Boc-Phe-Tyr-OH typically involves multiple steps, starting from readily available precursors. One common synthetic route includes the following steps:
Protection of the Hydroxy Group: The hydroxy group of the starting material is protected using a suitable protecting group, such as tert-butyl dimethylsilyl (TBDMS) chloride, to prevent unwanted reactions.
Formation of the Amide Bond: The protected hydroxy compound is then reacted with an appropriate amine to form an amide bond. This step often requires coupling reagents like N,N’-dicyclohexylcarbodiimide (DCC) and 4-dimethylaminopyridine (DMAP).
Deprotection: The protecting group is removed under mild conditions to yield the desired compound.
Industrial Production Methods
Industrial production of this compound may involve similar synthetic routes but on a larger scale. The use of automated reactors and continuous flow systems can enhance the efficiency and yield of the synthesis. Additionally, purification techniques such as crystallization, chromatography, and recrystallization are employed to obtain the compound in high purity.
Chemical Reactions Analysis
Types of Reactions
Boc-Phe-Tyr-OH undergoes various chemical reactions, including:
Oxidation: The hydroxy group can be oxidized to form a ketone or aldehyde using oxidizing agents like potassium permanganate (KMnO4) or chromium trioxide (CrO3).
Reduction: The compound can be reduced to form alcohols or amines using reducing agents such as lithium aluminum hydride (LiAlH4) or sodium borohydride (NaBH4).
Substitution: The hydroxy group can be substituted with other functional groups using reagents like thionyl chloride (SOCl2) or phosphorus tribromide (PBr3).
Common Reagents and Conditions
Oxidation: KMnO4, CrO3, H2O2
Reduction: LiAlH4, NaBH4, H2/Pd-C
Substitution: SOCl2, PBr3, NaH
Major Products
The major products formed from these reactions depend on the specific reagents and conditions used. For example, oxidation with KMnO4 can yield a ketone, while reduction with LiAlH4 can produce an alcohol.
Scientific Research Applications
Chemistry
In chemistry, Boc-Phe-Tyr-OH is used as a building block for the synthesis of more complex molecules. Its functional groups allow for diverse chemical modifications, making it valuable in the development of new materials and catalysts.
Biology
In biological research, this compound is studied for its potential as a biochemical probe. Its ability to interact with specific enzymes and receptors makes it useful in elucidating biological pathways and mechanisms.
Medicine
In medicine, this compound is investigated for its therapeutic potential. It may serve as a lead compound for the development of new drugs targeting specific diseases.
Industry
In the industrial sector, this compound is used in the production of specialty chemicals and advanced materials. Its unique properties make it suitable for applications in coatings, adhesives, and polymers.
Comparison with Similar Compounds
Similar Compounds
(2S)-3-(4-Hydroxyphenyl)-2-[[(2S)-2-amino-3-phenylpropanoyl]amino]propanoic acid: This compound lacks the tert-butoxycarbonyl group, which may affect its reactivity and biological activity.
(2S)-3-(4-Hydroxyphenyl)-2-[[(2S)-2-[(2-methylpropan-2-yl)oxycarbonylamino]-3-(4-hydroxyphenyl)propanoyl]amino]propanoic acid: This compound has an additional hydroxyphenyl group, which can influence its chemical and biological properties.
Uniqueness
The presence of the tert-butoxycarbonyl group in Boc-Phe-Tyr-OH imparts unique steric and electronic effects, making it distinct from similar compounds. These effects can enhance its stability, reactivity, and specificity in various applications.
Properties
IUPAC Name |
(2S)-3-(4-hydroxyphenyl)-2-[[(2S)-2-[(2-methylpropan-2-yl)oxycarbonylamino]-3-phenylpropanoyl]amino]propanoic acid |
Source
|
---|---|---|
Source | PubChem | |
URL | https://pubchem.ncbi.nlm.nih.gov | |
Description | Data deposited in or computed by PubChem | |
InChI |
InChI=1S/C23H28N2O6/c1-23(2,3)31-22(30)25-18(13-15-7-5-4-6-8-15)20(27)24-19(21(28)29)14-16-9-11-17(26)12-10-16/h4-12,18-19,26H,13-14H2,1-3H3,(H,24,27)(H,25,30)(H,28,29)/t18-,19-/m0/s1 |
Source
|
Source | PubChem | |
URL | https://pubchem.ncbi.nlm.nih.gov | |
Description | Data deposited in or computed by PubChem | |
InChI Key |
SIRXGPIISDLXFD-OALUTQOASA-N |
Source
|
Source | PubChem | |
URL | https://pubchem.ncbi.nlm.nih.gov | |
Description | Data deposited in or computed by PubChem | |
Canonical SMILES |
CC(C)(C)OC(=O)NC(CC1=CC=CC=C1)C(=O)NC(CC2=CC=C(C=C2)O)C(=O)O |
Source
|
Source | PubChem | |
URL | https://pubchem.ncbi.nlm.nih.gov | |
Description | Data deposited in or computed by PubChem | |
Isomeric SMILES |
CC(C)(C)OC(=O)N[C@@H](CC1=CC=CC=C1)C(=O)N[C@@H](CC2=CC=C(C=C2)O)C(=O)O |
Source
|
Source | PubChem | |
URL | https://pubchem.ncbi.nlm.nih.gov | |
Description | Data deposited in or computed by PubChem | |
Molecular Formula |
C23H28N2O6 |
Source
|
Source | PubChem | |
URL | https://pubchem.ncbi.nlm.nih.gov | |
Description | Data deposited in or computed by PubChem | |
Molecular Weight |
428.5 g/mol |
Source
|
Source | PubChem | |
URL | https://pubchem.ncbi.nlm.nih.gov | |
Description | Data deposited in or computed by PubChem | |
Disclaimer and Information on In-Vitro Research Products
Please be aware that all articles and product information presented on BenchChem are intended solely for informational purposes. The products available for purchase on BenchChem are specifically designed for in-vitro studies, which are conducted outside of living organisms. In-vitro studies, derived from the Latin term "in glass," involve experiments performed in controlled laboratory settings using cells or tissues. It is important to note that these products are not categorized as medicines or drugs, and they have not received approval from the FDA for the prevention, treatment, or cure of any medical condition, ailment, or disease. We must emphasize that any form of bodily introduction of these products into humans or animals is strictly prohibited by law. It is essential to adhere to these guidelines to ensure compliance with legal and ethical standards in research and experimentation.