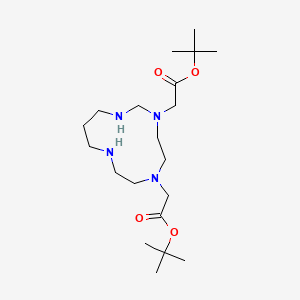
1,4-Bis(tert-butoxycarbonylmethyl)-1,4,7,10-tetraazacyclododecane
- Click on QUICK INQUIRY to receive a quote from our team of experts.
- With the quality product at a COMPETITIVE price, you can focus more on your research.
Overview
Description
1,4-Bis(tert-butoxycarbonylmethyl)-1,4,7,10-tetraazacyclododecane is a synthetic organic compound known for its unique chemical properties. It is characterized by the presence of four nitrogen atoms in a cyclic structure, with tert-butoxycarbonylmethyl groups attached to the 1 and 4 positions of the ring. This compound is often used as a ligand in coordination chemistry and has applications in various fields, including medicinal chemistry and materials science.
Preparation Methods
Synthetic Routes and Reaction Conditions
The synthesis of 1,4-Bis(tert-butoxycarbonylmethyl)-1,4,7,10-tetraazacyclododecane typically involves the reaction of 1,4,7,10-tetraazacyclododecane with tert-butyl bromoacetate in the presence of a base such as sodium hydride. The reaction is carried out in an aprotic solvent like dimethylformamide (DMF) at elevated temperatures. The product is then purified using column chromatography to obtain the desired compound in high yield .
Industrial Production Methods
While specific industrial production methods for this compound are not widely documented, the general approach involves scaling up the laboratory synthesis. This includes optimizing reaction conditions, using larger reaction vessels, and employing continuous flow techniques to enhance efficiency and yield. Industrial production also focuses on ensuring the purity and consistency of the final product through rigorous quality control measures.
Chemical Reactions Analysis
Types of Reactions
1,4-Bis(tert-butoxycarbonylmethyl)-1,4,7,10-tetraazacyclododecane undergoes various chemical reactions, including:
Substitution Reactions: The tert-butoxycarbonyl groups can be substituted with other functional groups using nucleophilic substitution reactions.
Complexation Reactions: The compound acts as a ligand and forms stable complexes with metal ions such as iron(II) and cobalt(II).
Common Reagents and Conditions
Nucleophilic Substitution: Reagents such as sodium hydride and alkyl halides are commonly used.
Complexation: Metal salts like iron(II) chloride and cobalt(II) chloride are used in the presence of the ligand under mild conditions.
Major Products Formed
Substitution Products: Various substituted derivatives depending on the nucleophile used.
Metal Complexes:
Scientific Research Applications
1,4-Bis(tert-butoxycarbonylmethyl)-1,4,7,10-tetraazacyclododecane has several scientific research applications:
Coordination Chemistry: Used as a ligand to form stable complexes with transition metals, which are studied for their catalytic properties.
Medicinal Chemistry: Investigated for its potential use in drug delivery systems and as a building block for pharmaceuticals.
Materials Science: Employed in the synthesis of advanced materials with specific electronic and magnetic properties.
Mechanism of Action
The mechanism of action of 1,4-Bis(tert-butoxycarbonylmethyl)-1,4,7,10-tetraazacyclododecane primarily involves its ability to act as a ligand and form stable complexes with metal ions. The nitrogen atoms in the cyclic structure coordinate with the metal ions, stabilizing them and enhancing their reactivity. This property is exploited in various catalytic processes and in the development of new materials .
Comparison with Similar Compounds
Similar Compounds
1,4,7,10-Tetraazacyclododecane: The parent compound without the tert-butoxycarbonyl groups.
1,4,7,10-Tetraazacyclododecane-1,4-diacetic acid: A derivative with acetic acid groups instead of tert-butoxycarbonyl groups.
Uniqueness
1,4-Bis(tert-butoxycarbonylmethyl)-1,4,7,10-tetraazacyclododecane is unique due to the presence of tert-butoxycarbonyl groups, which enhance its solubility and stability. This makes it more versatile in various chemical reactions and applications compared to its parent compound and other derivatives .
Biological Activity
1,4-Bis(tert-butoxycarbonylmethyl)-1,4,7,10-tetraazacyclododecane, also known as DOTA-tris(t-Bu ester), is a compound of significant interest in the fields of medicinal chemistry and biochemistry due to its properties as a bifunctional chelator. This article explores the biological activity of this compound, focusing on its synthesis, applications in medical imaging, and its interactions at the cellular level.
Chemical Structure and Properties
This compound is derived from cyclen (1,4,7,10-tetraazacyclododecane) and is characterized by the presence of tert-butoxycarbonyl groups. Its chemical formula is C26H50N4O6 and it has a molecular weight of 478.68 g/mol. The compound's structure allows it to form stable complexes with various metal ions, making it useful in a range of applications.
Synthesis
The synthesis of this compound typically involves the alkylation of cyclen with tert-butyl bromoacetate under controlled conditions. The reaction yields a product that can be purified through simple column chromatography. This method has been reported to provide high yields and good regioselectivity for the desired product .
1. Metal Chelation and Imaging Applications
One of the primary biological activities of this compound is its ability to chelate metal ions such as gadolinium(III). This property is exploited in medical imaging techniques like Magnetic Resonance Imaging (MRI). The gadolinium complexes formed with DOTA derivatives serve as contrast agents that enhance imaging quality .
Table 1: Comparison of Imaging Agents
Compound | Metal Ion | Application | Stability |
---|---|---|---|
DOTA-tris(t-Bu ester) | Gadolinium(III) | MRI Contrast Agent | High |
NODAGA | Copper(II) | PET Imaging | Moderate |
DO3A | Gallium(III) | Radiotherapy | High |
2. Cellular Uptake and Localization
Research indicates that DOTA-based compounds can be effectively internalized by cells. For instance, studies have shown that lanthanide complexes derived from DOTA can localize within the cell nucleus of NlH/3T3 cells. This internalization is crucial for applications in targeted drug delivery systems and photodynamic therapy .
Case Study 1: MRI Contrast Enhancement
In a study evaluating the efficacy of gadolinium-DOTA complexes in MRI imaging, it was found that these complexes provided significantly improved contrast in imaging soft tissues compared to traditional agents. The study highlighted the importance of the stability and kinetic inertness of these complexes in achieving reliable imaging results .
Case Study 2: Photodynamic Therapy
Another investigation focused on the application of DOTA derivatives in photodynamic therapy (PDT). The study demonstrated that upon activation with near-infrared light, these compounds could generate reactive oxygen species (ROS), leading to enhanced cytotoxic effects against cancer cells. This dual functionality—imaging and therapeutic—illustrates the versatility of DOTA derivatives in biomedical applications .
Properties
Molecular Formula |
C20H40N4O4 |
---|---|
Molecular Weight |
400.6 g/mol |
IUPAC Name |
tert-butyl 2-[3-[2-[(2-methylpropan-2-yl)oxy]-2-oxoethyl]-1,3,6,9-tetrazacyclododec-6-yl]acetate |
InChI |
InChI=1S/C20H40N4O4/c1-19(2,3)27-17(25)14-23-11-10-21-8-7-9-22-16-24(13-12-23)15-18(26)28-20(4,5)6/h21-22H,7-16H2,1-6H3 |
InChI Key |
DDQMYTWCHASSLF-UHFFFAOYSA-N |
Canonical SMILES |
CC(C)(C)OC(=O)CN1CCNCCCNCN(CC1)CC(=O)OC(C)(C)C |
Origin of Product |
United States |
Disclaimer and Information on In-Vitro Research Products
Please be aware that all articles and product information presented on BenchChem are intended solely for informational purposes. The products available for purchase on BenchChem are specifically designed for in-vitro studies, which are conducted outside of living organisms. In-vitro studies, derived from the Latin term "in glass," involve experiments performed in controlled laboratory settings using cells or tissues. It is important to note that these products are not categorized as medicines or drugs, and they have not received approval from the FDA for the prevention, treatment, or cure of any medical condition, ailment, or disease. We must emphasize that any form of bodily introduction of these products into humans or animals is strictly prohibited by law. It is essential to adhere to these guidelines to ensure compliance with legal and ethical standards in research and experimentation.