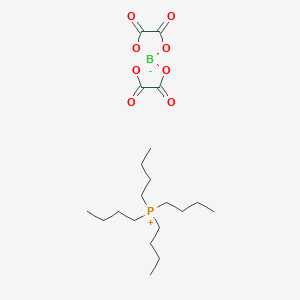
Tetra-n-butylphosphonium bis(oxalato-(2)-borate
- Click on QUICK INQUIRY to receive a quote from our team of experts.
- With the quality product at a COMPETITIVE price, you can focus more on your research.
Overview
Description
Tetra-n-butylphosphonium bis(oxalato-(2)-borate) is an ionic liquid that has garnered attention due to its unique properties and potential applications in various fields. This compound is composed of a tetra-n-butylphosphonium cation and a bis(oxalato-(2)-borate) anion. Ionic liquids like this one are known for their low volatility, high thermal stability, and ability to dissolve a wide range of substances, making them valuable in both academic research and industrial applications.
Preparation Methods
Synthetic Routes and Reaction Conditions
The synthesis of tetra-n-butylphosphonium bis(oxalato-(2)-borate) typically involves a metathesis reaction. One common method is to react tetra-n-butylphosphonium bromide with potassium bis(oxalato-(2)-borate) in an aqueous medium. The reaction proceeds as follows:
(C4H9)4PBr+K2B(C2O4)2→(C4H9)4PB(C2O4)2+2KBr
The reaction mixture is stirred at room temperature until the formation of the desired ionic liquid is complete. The product is then purified by washing with water to remove the potassium bromide byproduct and dried under vacuum.
Industrial Production Methods
On an industrial scale, the production of this compound) follows similar principles but is optimized for larger quantities. Continuous flow reactors and automated systems are often employed to ensure consistent quality and yield. The use of high-purity starting materials and stringent control of reaction conditions are crucial to achieving the desired product specifications.
Chemical Reactions Analysis
Types of Reactions
Tetra-n-butylphosphonium bis(oxalato-(2)-borate) can undergo various chemical reactions, including:
Substitution Reactions: The phosphonium cation can participate in nucleophilic substitution reactions.
Complexation Reactions: The oxalato borate anion can form complexes with metal ions.
Redox Reactions: The compound can be involved in redox reactions, particularly in electrochemical applications.
Common Reagents and Conditions
Nucleophilic Substitution: Reagents such as alkyl halides can react with the phosphonium cation under mild conditions.
Complexation: Metal salts like copper(II) sulfate can form complexes with the ox
Properties
Molecular Formula |
C20H36BO8P |
---|---|
Molecular Weight |
446.3 g/mol |
IUPAC Name |
tetrabutylphosphanium;1,4,6,9-tetraoxa-5-boranuidaspiro[4.4]nonane-2,3,7,8-tetrone |
InChI |
InChI=1S/C16H36P.C4BO8/c1-5-9-13-17(14-10-6-2,15-11-7-3)16-12-8-4;6-1-2(7)11-5(10-1)12-3(8)4(9)13-5/h5-16H2,1-4H3;/q+1;-1 |
InChI Key |
KZUMSUSIFDQSEN-UHFFFAOYSA-N |
Canonical SMILES |
[B-]12(OC(=O)C(=O)O1)OC(=O)C(=O)O2.CCCC[P+](CCCC)(CCCC)CCCC |
Origin of Product |
United States |
Disclaimer and Information on In-Vitro Research Products
Please be aware that all articles and product information presented on BenchChem are intended solely for informational purposes. The products available for purchase on BenchChem are specifically designed for in-vitro studies, which are conducted outside of living organisms. In-vitro studies, derived from the Latin term "in glass," involve experiments performed in controlled laboratory settings using cells or tissues. It is important to note that these products are not categorized as medicines or drugs, and they have not received approval from the FDA for the prevention, treatment, or cure of any medical condition, ailment, or disease. We must emphasize that any form of bodily introduction of these products into humans or animals is strictly prohibited by law. It is essential to adhere to these guidelines to ensure compliance with legal and ethical standards in research and experimentation.