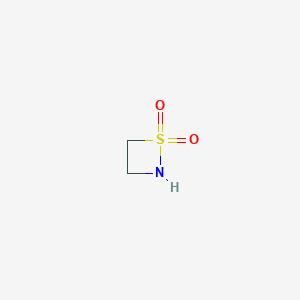
1,2-Thiazetidine 1,1-dioxide
Overview
Description
1,2-Thiazetidine 1,1-dioxide: is an organic compound with the molecular formula C₂H₅NO₂S. It is a cyclic sulfonamide that consists of a five-membered ring containing a nitrogen and sulfur atom, with an oxygen atom attached to the sulfur atom. . It is often used as a building block in organic synthesis due to its unique structural properties.
Mechanism of Action
Target of Action
The primary target of 1,2-Thiazetidine 1,1-dioxide is amino acids and dipeptides . The compound interacts with these targets, leading to the formation of N-silylated β-sultam peptides .
Mode of Action
This compound interacts with its targets through a process of condensation. Specifically, (RS)-2-tert-Butyldimethylsilyl-1,2-thiazetidine-3-acetic acid 1,1-dioxide, prepared from (RS)-S-benzyl-β-homocysteine, is condensed with various L-amino acid esters or dipeptide esters, yielding N-silylated β-sultam peptides . A β-sultam active ester is isolated as an intermediate in this process .
Biochemical Pathways
The biochemical pathways affected by this compound involve the formation of β-sultam peptides. These peptides are formed through the condensation of the compound with various L-amino acid esters or dipeptide esters
Result of Action
The primary result of the action of this compound is the formation of N-silylated β-sultam peptides . These peptides are formed through the condensation of the compound with various L-amino acid esters or dipeptide esters .
Action Environment
The action of this compound is influenced by environmental factors. For instance, the β-sultam peptides formed by the compound are sensitive to humidity, undergoing hydrolysis to form sulfonic acids . Additionally, reactions with amines result in the formation of sulfonamides . This suggests that the compound’s action, efficacy, and stability can be significantly affected by the surrounding environment.
Biochemical Analysis
Biochemical Properties
1,2-Thiazetidine 1,1-dioxide plays a significant role in biochemical reactions. It interacts with various enzymes, proteins, and other biomolecules. For instance, it has been observed that this compound can be N-alkylated using bromoacetates and N-acylated using acyl chlorides or protected amino acid fluorides . These interactions lead to the formation of β-sultam peptides, which are sensitive to humidity and hydrolyze to form sulfonic acids . Additionally, reactions with amines result in the formation of sulfonamides .
Cellular Effects
This compound has various effects on different types of cells and cellular processes. It influences cell function by interacting with cell signaling pathways, gene expression, and cellular metabolism. For example, the compound’s interaction with amino acids and dipeptides can lead to the formation of stable N-unsubstituted products . These interactions can affect cellular metabolism and gene expression, leading to changes in cell function.
Molecular Mechanism
The molecular mechanism of this compound involves its binding interactions with biomolecules, enzyme inhibition or activation, and changes in gene expression. The compound can be N-alkylated and N-acylated, leading to the formation of β-sultam peptides . These peptides can interact with various enzymes and proteins, leading to enzyme inhibition or activation. Additionally, the compound’s interaction with amino acids and dipeptides can result in changes in gene expression .
Temporal Effects in Laboratory Settings
In laboratory settings, the effects of this compound can change over time. The compound’s stability and degradation are important factors to consider. For instance, β-sultam peptides formed from this compound are sensitive to humidity and can hydrolyze to form sulfonic acids . Over time, these changes can affect the compound’s long-term effects on cellular function in both in vitro and in vivo studies.
Dosage Effects in Animal Models
The effects of this compound can vary with different dosages in animal models. At lower doses, the compound may have minimal effects, while at higher doses, it may exhibit toxic or adverse effects. For example, high doses of this compound can lead to enzyme inhibition or activation, resulting in changes in cellular metabolism and gene expression . These dosage-dependent effects are crucial for understanding the compound’s safety and efficacy in animal models.
Metabolic Pathways
This compound is involved in various metabolic pathways. It interacts with enzymes and cofactors, leading to changes in metabolic flux and metabolite levels. For instance, the compound can be N-alkylated and N-acylated, resulting in the formation of β-sultam peptides . These peptides can further interact with enzymes and proteins, affecting metabolic pathways and metabolite levels.
Transport and Distribution
The transport and distribution of this compound within cells and tissues are essential for understanding its biochemical effects. The compound can interact with transporters and binding proteins, affecting its localization and accumulation. For example, the formation of β-sultam peptides from this compound can influence its transport and distribution within cells . These interactions can affect the compound’s overall biochemical activity and function.
Subcellular Localization
The subcellular localization of this compound is crucial for its activity and function. The compound can be directed to specific compartments or organelles through targeting signals or post-translational modifications. For instance, the formation of β-sultam peptides from this compound can influence its subcellular localization . These peptides can be directed to specific cellular compartments, affecting the compound’s overall activity and function.
Preparation Methods
Synthetic Routes and Reaction Conditions: 1,2-Thiazetidine 1,1-dioxide can be synthesized from (RS)-S-benzyl-β-homocysteine through oxidative chlorination followed by ring closure with ammonia and silylation . The acid form can be prepared by hydrogenolysis in the presence of palladium on carbon . Another method involves N-alkylation using bromoacetates and N-acylation using acyl chlorides, protected amino acid fluorides, or N-protected amino acid NCA .
Industrial Production Methods: Industrial production methods for this compound are not well-documented in the literature. the synthetic routes mentioned above can be adapted for larger-scale production with appropriate optimization of reaction conditions and purification processes.
Chemical Reactions Analysis
Types of Reactions: 1,2-Thiazetidine 1,1-dioxide undergoes various chemical reactions, including:
Oxidation: The sulfonamide group can be oxidized to form sulfonic acids.
Reduction: Reduction reactions can convert the sulfonamide group to sulfonamides.
Substitution: The compound can undergo substitution reactions with various nucleophiles.
Common Reagents and Conditions:
Oxidation: Common oxidizing agents include hydrogen peroxide and peracids.
Reduction: Reducing agents such as lithium aluminum hydride can be used.
Substitution: Nucleophiles like amines and alcohols can react with the compound under basic or acidic conditions.
Major Products Formed:
Oxidation: Sulfonic acids.
Reduction: Sulfonamides.
Substitution: Various substituted derivatives depending on the nucleophile used.
Scientific Research Applications
1,2-Thiazetidine 1,1-dioxide has several scientific research applications:
Chemistry: It is used as a building block in the synthesis of more complex molecules, including β-sultam peptides.
Biology: The compound’s derivatives are studied for their potential biological activities.
Medicine: Research is ongoing to explore its potential as a pharmaceutical intermediate.
Industry: It is used in the development of new materials and chemical processes.
Comparison with Similar Compounds
1,2-Thiazetidine-3-acetic acid 1,1-dioxide: This compound is structurally similar but has an additional acetic acid group.
β-Sultam peptides: These are derivatives of 1,2-thiazetidine 1,1-dioxide and have similar reactivity.
Uniqueness: this compound is unique due to its cyclic sulfonamide structure, which imparts distinct chemical reactivity compared to other sulfonamides and lactams. Its ability to form stable N-unsubstituted products and its reactivity with various nucleophiles make it a valuable compound in organic synthesis .
Properties
IUPAC Name |
thiazetidine 1,1-dioxide | |
---|---|---|
Source | PubChem | |
URL | https://pubchem.ncbi.nlm.nih.gov | |
Description | Data deposited in or computed by PubChem | |
InChI |
InChI=1S/C2H5NO2S/c4-6(5)2-1-3-6/h3H,1-2H2 | |
Source | PubChem | |
URL | https://pubchem.ncbi.nlm.nih.gov | |
Description | Data deposited in or computed by PubChem | |
InChI Key |
GNPXXOFTVXIOLF-UHFFFAOYSA-N | |
Source | PubChem | |
URL | https://pubchem.ncbi.nlm.nih.gov | |
Description | Data deposited in or computed by PubChem | |
Canonical SMILES |
C1CS(=O)(=O)N1 | |
Source | PubChem | |
URL | https://pubchem.ncbi.nlm.nih.gov | |
Description | Data deposited in or computed by PubChem | |
Molecular Formula |
C2H5NO2S | |
Source | PubChem | |
URL | https://pubchem.ncbi.nlm.nih.gov | |
Description | Data deposited in or computed by PubChem | |
DSSTOX Substance ID |
DTXSID40514030 | |
Record name | 1lambda~6~,2-Thiazetidine-1,1-dione | |
Source | EPA DSSTox | |
URL | https://comptox.epa.gov/dashboard/DTXSID40514030 | |
Description | DSSTox provides a high quality public chemistry resource for supporting improved predictive toxicology. | |
Molecular Weight |
107.13 g/mol | |
Source | PubChem | |
URL | https://pubchem.ncbi.nlm.nih.gov | |
Description | Data deposited in or computed by PubChem | |
CAS No. |
34817-61-3 | |
Record name | 1lambda~6~,2-Thiazetidine-1,1-dione | |
Source | EPA DSSTox | |
URL | https://comptox.epa.gov/dashboard/DTXSID40514030 | |
Description | DSSTox provides a high quality public chemistry resource for supporting improved predictive toxicology. | |
Record name | 1,2-thiazetidine-1,1-dione | |
Source | European Chemicals Agency (ECHA) | |
URL | https://echa.europa.eu/information-on-chemicals | |
Description | The European Chemicals Agency (ECHA) is an agency of the European Union which is the driving force among regulatory authorities in implementing the EU's groundbreaking chemicals legislation for the benefit of human health and the environment as well as for innovation and competitiveness. | |
Explanation | Use of the information, documents and data from the ECHA website is subject to the terms and conditions of this Legal Notice, and subject to other binding limitations provided for under applicable law, the information, documents and data made available on the ECHA website may be reproduced, distributed and/or used, totally or in part, for non-commercial purposes provided that ECHA is acknowledged as the source: "Source: European Chemicals Agency, http://echa.europa.eu/". Such acknowledgement must be included in each copy of the material. ECHA permits and encourages organisations and individuals to create links to the ECHA website under the following cumulative conditions: Links can only be made to webpages that provide a link to the Legal Notice page. | |
Retrosynthesis Analysis
AI-Powered Synthesis Planning: Our tool employs the Template_relevance Pistachio, Template_relevance Bkms_metabolic, Template_relevance Pistachio_ringbreaker, Template_relevance Reaxys, Template_relevance Reaxys_biocatalysis model, leveraging a vast database of chemical reactions to predict feasible synthetic routes.
One-Step Synthesis Focus: Specifically designed for one-step synthesis, it provides concise and direct routes for your target compounds, streamlining the synthesis process.
Accurate Predictions: Utilizing the extensive PISTACHIO, BKMS_METABOLIC, PISTACHIO_RINGBREAKER, REAXYS, REAXYS_BIOCATALYSIS database, our tool offers high-accuracy predictions, reflecting the latest in chemical research and data.
Strategy Settings
Precursor scoring | Relevance Heuristic |
---|---|
Min. plausibility | 0.01 |
Model | Template_relevance |
Template Set | Pistachio/Bkms_metabolic/Pistachio_ringbreaker/Reaxys/Reaxys_biocatalysis |
Top-N result to add to graph | 6 |
Feasible Synthetic Routes
Q1: What are β-sultams?
A1: β-sultams are four-membered cyclic sulfonamides formally derived from the hypothetical parent compound 1,2-thiazetidine 1,1-dioxide. These compounds are structurally analogous to β-lactams but contain a sulfur atom instead of a carbon atom at the 1-position in the ring.
Q2: How are 1,2-Thiazetidine 1,1-dioxides synthesized?
A2: Several synthetic routes have been explored for this compound synthesis. These include:
- Cyclization of 2-Alkylethenesulfonyl Fluorides: Reacting β-alkylethenesulfonic acid fluorides with primary amines yields 2,3-dialkyl 1,2-thiazetidine 1,1-dioxides. []
- Reactions of Sulfenes with Schiff Bases: Phenylsulfene generated from benzylsulfonyl chloride and triethylamine reacts with N-(p-substituted)-benzylidene-methylamine to give 2-methyl-4-phenyl-3-(p-substituted-phenyl)-1,2-thiazetidine 1,1-dioxide. []
- Pentafluorophenyl Sulfonates: A stereoselective one-pot synthesis utilizes heterocyclic pentafluorophenyl sulfonates, where N-O bond cleavage of isoxazolidines is followed by intramolecular cyclization. [, ]
Q3: What is the stereochemistry of 4-alkylated 1,2-Thiazetidine 1,1-dioxides?
A3: The synthesis of 4-alkylated derivatives often results in cis and trans isomers, which can be determined through ¹H-NMR and NOE difference spectroscopy. []
Q4: What are the key structural features of 1,2-Thiazetidine 1,1-dioxides?
A4: 1,2-Thiazetidine 1,1-dioxides possess a strained four-membered ring structure containing a sulfonyl group (SO2) adjacent to the nitrogen atom. The ring strain and the presence of the electron-withdrawing sulfonyl group contribute significantly to their reactivity. [, ]
Q5: How do 1,2-Thiazetidine 1,1-dioxides react with organolithium reagents?
A5: Treatment with organolithium reagents like butyllithium can induce dimerization and rearrangement, leading to the formation of six-membered heterocycles. []
Q6: Can 1,2-Thiazetidine 1,1-dioxides undergo hydrolysis?
A6: Yes, hydrolysis of this compound can lead to two potential products: N-ethyl amino-methyl sulfonate (P1) and 2-taurine methyl ester (P2). Water-assisted hydrolysis significantly lowers the energy barriers compared to non-assisted pathways. [, ]
Q7: How do solvents influence the hydrolysis of this compound?
A7: Solvent effects, particularly in polar solvents, can be modeled using the Polarizable Continuum Model (PCM). [, ]
Q8: What is the impact of silyl protecting groups on the reactivity of 1,2-Thiazetidine 1,1-dioxides?
A8: Silyl protecting groups can be strategically employed to control the regioselectivity of reactions. For example, N-silylated derivatives undergo acylation at C-4, while 2,3-disubstituted β-sultams are selectively silylated at C-4. [, ] The silyl group can be easily removed using tetrabutylammonium fluoride (TBAF). []
Q9: Can 1,2-Thiazetidine 1,1-dioxides undergo ring transformations?
A9: Yes, Lewis acids can induce ring transformations in 1,2-thiazetidine 1,1-dioxides. This can lead to the formation of trans-1,2,3-oxathiazolidine 2-oxides and cis-aziridines. []
Q10: Have computational methods been used to study 1,2-Thiazetidine 1,1-dioxides?
A10: Yes, both ab initio and density functional theory (DFT) calculations, particularly at HF/6-31G, MP2/6-31G, and B3LYP/6-31G* levels, have been employed to investigate the hydrolysis mechanism of this compound. [, ] DFT studies have also explored the effects of water and alcohol on the alcoholysis mechanisms. []
Q11: What is known about the structure-activity relationships (SAR) of 1,2-Thiazetidine 1,1-dioxides?
A11: SAR studies are crucial to understanding the relationship between the structure of 1,2-Thiazetidine 1,1-dioxides and their biological activity. While detailed SAR data is limited in the provided research, it's understood that modifications at different positions of the ring can significantly impact their reactivity, stability, and potential biological activities. [, , ]
Disclaimer and Information on In-Vitro Research Products
Please be aware that all articles and product information presented on BenchChem are intended solely for informational purposes. The products available for purchase on BenchChem are specifically designed for in-vitro studies, which are conducted outside of living organisms. In-vitro studies, derived from the Latin term "in glass," involve experiments performed in controlled laboratory settings using cells or tissues. It is important to note that these products are not categorized as medicines or drugs, and they have not received approval from the FDA for the prevention, treatment, or cure of any medical condition, ailment, or disease. We must emphasize that any form of bodily introduction of these products into humans or animals is strictly prohibited by law. It is essential to adhere to these guidelines to ensure compliance with legal and ethical standards in research and experimentation.