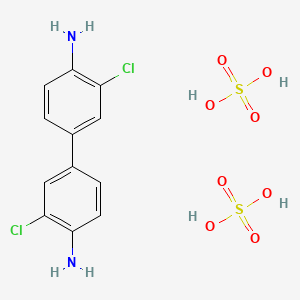
3,3'-Dichlorobenzidine sulfate
- Click on QUICK INQUIRY to receive a quote from our team of experts.
- With the quality product at a COMPETITIVE price, you can focus more on your research.
Overview
Description
3,3’-Dichlorobenzidine sulfate is an organic compound with the formula (C₆H₃Cl(NH₂))₂·H₂SO₄. It is a pale yellow crystalline solid that is barely soluble in water. This compound is primarily used in the production of diarylide yellow pigments, which are utilized in printing inks . its use has been largely discontinued due to concerns about its carcinogenicity .
Preparation Methods
3,3’-Dichlorobenzidine sulfate is synthesized from 2-nitrochlorobenzene through a two-step process. The first step involves the reduction of 2-nitrochlorobenzene with zinc dust and sodium hydroxide to produce 2,2’-dichlorohydrazobenzene . This intermediate then undergoes a benzidine rearrangement in the presence of hydrochloric or sulfuric acid to yield 3,3’-dichlorobenzidine . The sulfate salt is formed by treating 3,3’-dichlorobenzidine with sulfuric acid .
Chemical Reactions Analysis
3,3’-Dichlorobenzidine sulfate undergoes several types of chemical reactions:
Oxidation: It can be oxidized to form tetrachloro derivatives.
Reduction: The initial reduction step in its synthesis involves the reduction of 2-nitrochlorobenzene.
Common reagents used in these reactions include zinc dust, sodium hydroxide, hydrochloric acid, and sulfuric acid . Major products formed from these reactions include various yellow pigments such as Pigment Yellow 12, Pigment Yellow 13, Pigment Yellow 14, Pigment Yellow 17, and Pigment Yellow 83 .
Scientific Research Applications
3,3’-Dichlorobenzidine sulfate has several scientific research applications:
Chemistry: It is used as a starting material for the synthesis of diarylide pigments.
Environmental Science: It is studied for its environmental impact due to its carcinogenic nature.
Analytical Chemistry: It is used as an analytical standard in high-performance liquid chromatography (HPLC) and gas chromatography (GC) for the detection of similar compounds.
Mechanism of Action
The mechanism by which 3,3’-Dichlorobenzidine sulfate exerts its effects is primarily through its structural similarity to benzidine, a known human bladder carcinogen . It is believed to cause cancer by forming DNA adducts, leading to mutations and tumor formation . The compound’s molecular targets include DNA and various enzymes involved in DNA repair and replication .
Comparison with Similar Compounds
3,3’-Dichlorobenzidine sulfate is similar to other benzidine derivatives such as:
Benzidine: A known human bladder carcinogen with similar chemical structure and carcinogenic properties.
3,3’-Dichlorobenzidine dihydrochloride: Another salt form of 3,3’-dichlorobenzidine with similar toxicological properties.
3,3’-Dichlorobenzidine dihydrogen bis(sulfate): Another sulfate salt with similar chemical properties.
The uniqueness of 3,3’-Dichlorobenzidine sulfate lies in its specific use in the production of diarylide yellow pigments and its distinct carcinogenic profile .
Biological Activity
3,3'-Dichlorobenzidine sulfate (DCB sulfate) is a synthetic organic compound that has garnered attention due to its potential biological activities, particularly its mutagenic and carcinogenic properties. This article delves into the biological activity of DCB sulfate, supported by empirical data, case studies, and research findings.
This compound is a derivative of 3,3'-dichlorobenzidine (DCB), a compound known for its use in dye manufacturing and as a precursor in various chemical syntheses. The compound's structure includes two chlorine atoms attached to a benzidine moiety, which contributes to its reactivity and biological effects.
Upon exposure, DCB undergoes metabolic conversion primarily through cytochrome P450 enzymes, leading to the formation of reactive intermediates that can bind covalently to macromolecules such as DNA and proteins. These interactions are critical in understanding its mutagenic potential. Studies indicate that approximately 90% of DCB is metabolized into various conjugated metabolites that are excreted via urine within 72 hours post-exposure .
Mutagenicity and Genotoxicity
In Vitro Studies:
Research has demonstrated that DCB sulfate exhibits significant mutagenic activity. In vitro assays have shown that it can induce unscheduled DNA synthesis in human fibroblasts and increase the frequency of sister chromatid exchanges in various cell lines .
In Vivo Studies:
Animal studies provide further evidence of DCB's genotoxic effects. For instance, oral administration of DCB to rodents resulted in increased micronuclei formation in bone marrow cells, indicating chromosomal damage . Additionally, elevated levels of DNA adducts have been detected in the liver and bladder tissues of exposed animals .
Carcinogenic Potential
The U.S. Department of Health and Human Services classifies DCB as "reasonably anticipated to be a human carcinogen." Epidemiological studies have linked occupational exposure to DCB with increased incidences of bladder cancer and other malignancies . In laboratory settings, DCB has caused tumors in multiple organ sites among treated animals, reinforcing its classification as a probable human carcinogen .
Case Studies
-
Epidemiological Evidence:
A cohort study involving workers exposed to DCB revealed a statistically significant increase in bladder cancer cases compared to unexposed populations. This study highlights the long-term risks associated with chronic exposure to DCB . -
Animal Models:
In a controlled study, rats administered DCB at varying doses exhibited dose-dependent increases in liver tumors. The lowest-observed-adverse-effect level (LOAEL) was identified at doses as low as 500 mg/kg body weight .
Biological Monitoring
Biomarkers for exposure to DCB sulfate include urinary levels of the compound and its metabolites, as well as hemoglobin adducts formed during metabolic processing. These biomarkers serve as crucial tools for assessing human exposure levels and potential health risks associated with DCB .
Properties
CAS No. |
64969-34-2 |
---|---|
Molecular Formula |
C12H14Cl2N2O8S2 |
Molecular Weight |
449.3 g/mol |
IUPAC Name |
4-(4-amino-3-chlorophenyl)-2-chloroaniline;sulfuric acid |
InChI |
InChI=1S/C12H10Cl2N2.2H2O4S/c13-9-5-7(1-3-11(9)15)8-2-4-12(16)10(14)6-8;2*1-5(2,3)4/h1-6H,15-16H2;2*(H2,1,2,3,4) |
InChI Key |
PMZNABNRKYMIKT-UHFFFAOYSA-N |
Canonical SMILES |
C1=CC(=C(C=C1C2=CC(=C(C=C2)N)Cl)Cl)N.OS(=O)(=O)O.OS(=O)(=O)O |
physical_description |
3,3'-dichlorobenzidine sulfate appears as a white crystalline powder. Toxic by ingestion and skin absorption. |
Origin of Product |
United States |
Disclaimer and Information on In-Vitro Research Products
Please be aware that all articles and product information presented on BenchChem are intended solely for informational purposes. The products available for purchase on BenchChem are specifically designed for in-vitro studies, which are conducted outside of living organisms. In-vitro studies, derived from the Latin term "in glass," involve experiments performed in controlled laboratory settings using cells or tissues. It is important to note that these products are not categorized as medicines or drugs, and they have not received approval from the FDA for the prevention, treatment, or cure of any medical condition, ailment, or disease. We must emphasize that any form of bodily introduction of these products into humans or animals is strictly prohibited by law. It is essential to adhere to these guidelines to ensure compliance with legal and ethical standards in research and experimentation.