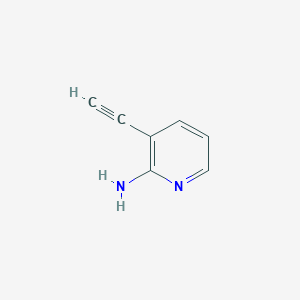
3-Ethynylpyridin-2-amine
Overview
Description
3-Ethynylpyridin-2-amine (CAS: 67346-74-1) is a pyridine derivative with the molecular formula C₇H₆N₂ and a molecular weight of 118.14 g/mol . It features an ethynyl group (-C≡CH) at the 3-position and an amino group (-NH₂) at the 2-position of the pyridine ring. This compound is primarily used in research settings, particularly in medicinal chemistry and materials science, due to its reactive ethynyl group, which enables click chemistry and metal-catalyzed coupling reactions .
Preparation Methods
Sonogashira Coupling with 3-Halo-2-Aminopyridines
General Procedure
The most widely reported method involves Sonogashira coupling between 3-halo-2-aminopyridines (X = Br, I) and terminal alkynes. Key steps include:
- Catalyst System : Pd(PPh₃)₂Cl₂ or Pd(PPh₃)₄ with CuI.
- Base : Triethylamine (Et₃N) or diisopropylamine.
- Solvent : Dichloromethane (DCM), acetonitrile, or tetrahydrofuran (THF).
- Alkyne Source : Ethynyltrimethylsilane (TMS-acetylene) or protected alkynes.
Example 1: Direct Coupling with TMS-Acetylene
Reagents :
- 3-Iodo-2-aminopyridine, Pd(PPh₃)₂Cl₂, CuI, TMS-acetylene, Et₃N.
Procedure :
1. React 3-iodo-2-aminopyridine with TMS-acetylene under Sonogashira conditions.
2. Deprotect TMS group using tetrabutylammonium fluoride (TBAF).
Yield : ~70–80% (estimated from analogous reactions).
Example 2: Coupling with 1,1-Dimethyl-2-Propyn-1-ol
Reagents :
- 3-Iodo-2-aminopyridine, Pd(PPh₃)₂Cl₂, CuI, 1,1-dimethyl-2-propyn-1-ol, Et₃N.
Procedure :
1. Perform Sonogashira coupling in DCM.
2. Hydrolyze the dimethyl-protected alkyne using LiOH/KOtBu.
Yield : 62% (reported in).
Optimization Parameters
Parameter | Optimal Conditions | Impact on Yield |
---|---|---|
Catalyst | Pd(PPh₃)₂Cl₂ (0.5–1 mol%) | High efficiency |
Solvent | DCM or THF | Minimal side reactions |
Temperature | 25–80°C (microwave-assisted) | Reduced reaction time |
Base | Et₃N or DIEA | Improved coupling efficiency |
Alternative Approaches
Halogenation Followed by Coupling
Step 1 : Iodination of 2-Aminopyridine
- Reagents : N-Iodosuccinimide (NIS), directed ortho-metalation.
- Yield : ~60–75% for 3-iodo-2-aminopyridine.
Step 2 : Sonogashira Coupling (as above).
Microwave-Assisted Synthesis
Conditions :
- Microwave irradiation (140°C, 1.25 h).
- Solvent : 1,4-Dioxane.
Advantage : 50% reduction in reaction time vs. conventional heating.
Challenges and Solutions
Challenge | Solution |
---|---|
Instability of 3-Iodo-2-aminopyridine | Use fresh reagents, inert atmosphere |
Low solubility in polar solvents | Employ DCM/THF mixtures |
Deprotection side reactions | Optimize TBAF stoichiometry |
Comparative Analysis of Methods
Method | Yield (%) | Purity (%) | Scalability |
---|---|---|---|
Sonogashira (TMS) | 70–80 | ≥95 | High |
Microwave-assisted | 65–75 | ≥90 | Moderate |
Deprotection (LiOH) | 60–70 | ≥85 | High |
Chemical Reactions Analysis
Types of Reactions: 3-Ethynylpyridin-2-amine can undergo various chemical reactions, including:
Oxidation: The ethynyl group can be oxidized to form carbonyl compounds.
Reduction: The pyridine ring can be reduced to form piperidine derivatives.
Substitution: The amino group can participate in nucleophilic substitution reactions.
Common Reagents and Conditions:
Oxidation: Reagents such as potassium permanganate or chromium trioxide can be used under acidic or basic conditions.
Reduction: Hydrogen gas in the presence of a palladium or platinum catalyst is commonly used.
Substitution: Halogenating agents like bromine or chlorine can be used to introduce halogen atoms into the molecule.
Major Products Formed:
Oxidation: Formation of pyridine-2-carboxaldehyde or pyridine-2-carboxylic acid.
Reduction: Formation of 3-ethynylpiperidine-2-amine.
Substitution: Formation of halogenated derivatives such as 3-bromo- or 3-chloropyridin-2-amine.
Scientific Research Applications
Medicinal Chemistry
Antimicrobial Activity
3-Ethynylpyridin-2-amine and its derivatives have demonstrated notable antimicrobial properties. Research has shown that compounds derived from this structure exhibit activity against various pathogens, including Cryptococcus neoformans, a yeast responsible for serious infections in immunocompromised individuals. Studies indicate that low concentrations of these compounds can effectively inhibit the growth of this pathogen, suggesting their potential as lead compounds for developing new antimicrobial agents.
Enzyme Inhibition
The compound has been investigated for its ability to modulate enzyme activity within biological systems. For instance, it has been identified as a potential inhibitor of enzymes involved in pathogen metabolism, which could pave the way for novel therapeutic strategies against infectious diseases. Additionally, its structural analogs have been explored for their selective inhibition of human neuronal nitric oxide synthase, highlighting its role in neurological research .
Material Science
Synthesis of Novel Materials
Due to its unique chemical structure, this compound can serve as a building block in the synthesis of new materials with specific electronic or optical properties. Researchers are exploring its applications in the development of polymers and nanomaterials that exhibit enhanced stability and functionality . The compound's reactivity allows for the creation of materials that can be tailored for specific industrial applications.
Nanotechnology Applications
The compound's properties are also being harnessed in the field of nanotechnology. For example, it has been utilized in the engineering of nanoparticles for biomedical applications, including drug delivery systems and biosensing technologies . The ability to modify its structure enables the design of nanoparticles that can selectively target specific biological pathways.
Biochemical Research
Biochemical Probes
this compound is being used as a biochemical probe to study various biological processes. Its derivatives are employed in assays to investigate enzyme interactions and cellular pathways, helping researchers understand complex biological mechanisms. This application is crucial for advancing our knowledge in fields such as pharmacology and toxicology.
Potential Therapeutic Applications
The compound's potential therapeutic effects extend beyond antimicrobial activity. Ongoing research is assessing its efficacy against various diseases, including cancer and neurodegenerative disorders. The ability to modulate key biological pathways suggests that this compound could contribute to developing new treatments for conditions that currently lack effective therapies .
Data Summary Table
Case Studies
-
Antimicrobial Efficacy Study
A study published in a peer-reviewed journal demonstrated that derivatives of this compound exhibited potent antifungal activity against Cryptococcus neoformans. The study highlighted the structure-activity relationship (SAR) that contributed to the observed biological activity, suggesting modifications that could enhance efficacy further. -
Polymer Development Research
Researchers have synthesized novel polymers incorporating this compound as a monomer. These polymers displayed unique optical properties suitable for applications in photonic devices, showcasing the compound's versatility beyond traditional uses. -
Neuroscience Application
A recent investigation into human neuronal nitric oxide synthase inhibitors revealed that modifications to the this compound scaffold significantly enhanced selectivity and potency. This research underscores the compound's potential in developing targeted therapies for neurological disorders.
Mechanism of Action
The mechanism of action of 3-Ethynylpyridin-2-amine involves its interaction with molecular targets such as enzymes and receptors. The ethynyl group can form covalent bonds with active sites of enzymes, inhibiting their activity. Additionally, the amino group can participate in hydrogen bonding and electrostatic interactions with receptor sites, modulating their function. These interactions can affect various biochemical pathways, leading to the compound’s observed effects.
Comparison with Similar Compounds
Key Properties :
- Solubility : Soluble in DMSO and other polar aprotic solvents.
- Storage : Stable at -80°C for 6 months or -20°C for 1 month.
- Purity : >97% (HPLC) in commercially available batches .
Comparison with Structurally Similar Compounds
5-Ethynylpyridin-2-amine
- Molecular Formula : C₇H₆N₂ (same as 3-ethynyl isomer).
- CAS : 82454-61-3.
- Key Differences: The ethynyl group is at the 5-position instead of the 3-position. Exhibits distinct electronic properties due to altered conjugation between the amino and ethynyl groups. Used in similar applications but may show divergent reactivity in cross-coupling reactions .
3-[(3-Chlorophenyl)ethynyl]pyridin-2-amine (11c)
- Molecular Formula : C₁₃H₉ClN₂.
- Synthesis: Prepared via Sonogashira coupling of 2-amino-3-bromopyridine with 1-ethynyl-3-chlorobenzene (86% yield) .
- Key Differences: Incorporates a chlorophenyl-ethynyl substituent, enhancing steric bulk and lipophilicity. Potential for enhanced biological activity due to the chlorine atom’s electron-withdrawing effects .
4-Methoxy-3-((trimethylsilyl)ethynyl)pyridin-2-amine
- Molecular Formula : C₁₂H₁₇N₂OSi.
- Key Differences :
2-(Thiophen-2-yl)pyridin-3-amine
- Molecular Formula : C₉H₈N₂S.
- Key Differences: Replaces the ethynyl group with a thiophene ring, introducing sulfur-based π-conjugation.
Structural and Functional Analysis
Electronic Effects
- 3-Ethynylpyridin-2-amine: The ethynyl group at the 3-position creates partial conjugation with the amino group, leading to moderate electron-withdrawing effects .
- Trimethylsilyl-protected analogs : The TMS group blocks unwanted side reactions while maintaining the ethynyl group’s utility in subsequent modifications .
Comparative Data Table
Compound Name | Molecular Formula | Molecular Weight | Substituents | Key Applications |
---|---|---|---|---|
This compound | C₇H₆N₂ | 118.14 | Ethynyl (3), NH₂ (2) | Click chemistry, drug design |
5-Ethynylpyridin-2-amine | C₇H₆N₂ | 118.14 | Ethynyl (5), NH₂ (2) | Materials science |
3-[(3-Chlorophenyl)ethynyl]pyridin-2-amine | C₁₃H₉ClN₂ | 228.68 | Ethynyl-Cl (3), NH₂ (2) | Antimicrobial studies |
4-Methoxy-3-(TMS-ethynyl)pyridin-2-amine | C₁₂H₁₇N₂OSi | 237.36 | TMS-ethynyl (3), OMe (4) | Protective group chemistry |
Research Implications
Biological Activity
3-Ethynylpyridin-2-amine is a compound that has garnered attention in the field of medicinal chemistry due to its potential biological activities. This article aims to summarize the current understanding of its biological properties, mechanisms of action, and applications in drug discovery.
Chemical Structure and Properties
This compound features an ethynyl group attached to the pyridine ring, which enhances its reactivity and allows for various chemical modifications. This structural characteristic is crucial for its interaction with biological targets.
The biological activity of this compound is closely related to its ability to interact with specific molecular targets, such as enzymes and receptors. The ethynyl group can facilitate nucleophilic substitutions and cross-coupling reactions, which are essential for synthesizing derivatives with enhanced biological profiles .
Antimicrobial Activity
Recent studies have indicated that derivatives of this compound exhibit significant antimicrobial properties. For instance, certain synthesized azaindoles derived from this compound showed potent activity against various bacteria and yeasts, particularly against Cryptococcus neoformans, with minimal inhibitory concentrations as low as 3.9 μg/ml .
Anticancer Potential
The compound has also been investigated for its anticancer properties. Research highlights that it may target specific pathways involved in tumor growth and angiogenesis. Integrin αvβ3, a key player in tumor neovascularization, has been identified as a potential target for compounds derived from this compound, suggesting its utility in designing antiangiogenic drugs .
Case Studies
- Synthesis and Evaluation of Derivatives : A study synthesized several derivatives of this compound through Sonogashira coupling reactions. These derivatives were tested for their inhibitory activity against nitric oxide synthases (NOS), revealing promising results with improved potency and selectivity .
- Antimicrobial Testing : In another study, various 7-azaindole derivatives synthesized from this compound were evaluated for their antimicrobial efficacy. The results demonstrated that these derivatives had significant activity against yeast strains, indicating the potential for developing new antifungal agents .
Data Summary
Biological Activity | Tested Against | Minimum Inhibitory Concentration (MIC) |
---|---|---|
Antimicrobial | Cryptococcus neoformans | 3.9 μg/ml |
Anticancer | Various cancer cell lines | Potent inhibition observed |
NOS Inhibition | Rat nNOS and human nNOS | Ki values: Rat nNOS (15 nM), Human nNOS (19 nM) |
Q & A
Q. What are the most reliable synthetic routes for 3-Ethynylpyridin-2-amine, and how can competing side reactions be minimized?
Basic Research Focus
The synthesis of this compound typically involves introducing an ethynyl group to a pyridine scaffold. One method involves Sonogashira coupling between 3-halopyridin-2-amine (e.g., 3-iodopyridin-2-amine) and trimethylsilylacetylene (TMSA), followed by deprotection of the TMS group under mild acidic conditions . Key considerations include:
- Catalytic system : Use Pd(PPh₃)₄/CuI for cross-coupling efficiency.
- Side reactions : Competing Glaser coupling (dimerization of alkynes) can be suppressed by degassing solvents and maintaining low temperatures (0–5°C).
- Purification : Column chromatography with silica gel (ethyl acetate/hexane, 1:3) yields >85% purity.
Advanced Consideration : For regioselective ethynylation, computational modeling (DFT) can predict electronic effects of substituents on pyridine ring reactivity .
Q. How can hydrogen-bonding interactions of this compound be characterized, and what role do they play in crystal packing?
Basic Research Focus
Single-crystal X-ray diffraction (SC-XRD) is the gold standard for resolving intermolecular interactions. For pyridine derivatives:
- Hydrogen bonding : The amine group (–NH₂) forms N–H⋯N hydrogen bonds with pyridine nitrogen atoms, creating centrosymmetric dimers .
- Cl⋯Cl interactions (if halogenated analogs exist): Observed at distances of ~3.28 Å, contributing to layered crystal packing .
Advanced Consideration : Hirshfeld surface analysis quantifies interaction contributions (e.g., H-bonding vs. van der Waals) and correlates with solubility parameters .
Q. What analytical techniques are critical for validating the purity and structure of this compound?
Basic Research Focus
- NMR spectroscopy :
- Mass spectrometry (HRMS) : Confirm molecular ion peaks (e.g., [M+H]⁺ for C₇H₇N₂: calc. 119.0609) .
Advanced Consideration : Use dynamic nuclear polarization (DNP) NMR to enhance sensitivity for trace impurities (<0.5%) .
Q. How does the ethynyl group influence the electronic properties and reactivity of this compound?
Advanced Research Focus
- Electronic effects : The ethynyl group is electron-withdrawing via conjugation, lowering the LUMO energy of the pyridine ring and enhancing electrophilic substitution at the 5-position .
- Reactivity in cross-coupling : Ethynyl groups enable further functionalization via click chemistry (e.g., CuAAC with azides) or Suzuki-Miyaura coupling with aryl boronic acids .
- DFT studies : Predict charge distribution and frontier molecular orbitals to guide reaction design .
Q. What safety protocols are essential for handling this compound in laboratory settings?
Basic Research Focus
- Personal protective equipment (PPE) : Use nitrile gloves, lab coats, and safety goggles.
- Ventilation : Perform reactions in a fume hood due to potential amine vapors .
- Spill management : Neutralize with 5% acetic acid and absorb with inert material (e.g., vermiculite) .
Advanced Consideration : Conduct thermal stability analysis (DSC/TGA) to assess decomposition risks under reaction conditions .
Q. What catalytic applications are possible for this compound-derived ligands?
Advanced Research Focus
- Coordination chemistry : The ethynyl group can anchor ligands to metal surfaces (e.g., Au nanoparticles) for heterogeneous catalysis .
- Homogeneous catalysis : Complexes with Pd or Ru catalyze C–H activation or hydrogenation reactions. Example: Pd(II)-ethynylpyridinamine complexes show 90% yield in Suzuki couplings .
- Ligand design : Modify the ethynyl group to tune steric/electronic effects (e.g., replace with ferrocenyl for redox-active catalysts) .
Q. How can contradictions in reported synthetic yields be resolved?
Advanced Research Focus
- Reproducibility analysis : Verify catalyst loading (e.g., Pd 2 mol% vs. 5 mol%), solvent purity, and reaction scale effects.
- In situ monitoring : Use Raman spectroscopy to track reaction progress and identify intermediates .
- Data reconciliation : Apply multivariate analysis (e.g., PCA) to isolate critical variables (temperature, base strength) from literature datasets .
Q. What biological screening strategies are suitable for this compound derivatives?
Advanced Research Focus
- Targeted assays : Screen against kinase enzymes (e.g., EGFR, VEGFR) due to pyridine’s affinity for ATP-binding pockets .
- ADMET profiling : Use in vitro models (Caco-2 for permeability, microsomes for metabolic stability) .
- Structural analogs : Compare with fluorinated pyridines (e.g., 3,5-difluoro-4-methylpyridin-2-amine) to assess bioavailability trends .
Properties
IUPAC Name |
3-ethynylpyridin-2-amine | |
---|---|---|
Source | PubChem | |
URL | https://pubchem.ncbi.nlm.nih.gov | |
Description | Data deposited in or computed by PubChem | |
InChI |
InChI=1S/C7H6N2/c1-2-6-4-3-5-9-7(6)8/h1,3-5H,(H2,8,9) | |
Source | PubChem | |
URL | https://pubchem.ncbi.nlm.nih.gov | |
Description | Data deposited in or computed by PubChem | |
InChI Key |
DQEVAYRCQIJNJE-UHFFFAOYSA-N | |
Source | PubChem | |
URL | https://pubchem.ncbi.nlm.nih.gov | |
Description | Data deposited in or computed by PubChem | |
Canonical SMILES |
C#CC1=C(N=CC=C1)N | |
Source | PubChem | |
URL | https://pubchem.ncbi.nlm.nih.gov | |
Description | Data deposited in or computed by PubChem | |
Molecular Formula |
C7H6N2 | |
Source | PubChem | |
URL | https://pubchem.ncbi.nlm.nih.gov | |
Description | Data deposited in or computed by PubChem | |
DSSTOX Substance ID |
DTXSID70497524 | |
Record name | 3-Ethynylpyridin-2-amine | |
Source | EPA DSSTox | |
URL | https://comptox.epa.gov/dashboard/DTXSID70497524 | |
Description | DSSTox provides a high quality public chemistry resource for supporting improved predictive toxicology. | |
Molecular Weight |
118.14 g/mol | |
Source | PubChem | |
URL | https://pubchem.ncbi.nlm.nih.gov | |
Description | Data deposited in or computed by PubChem | |
CAS No. |
67346-74-1 | |
Record name | 3-Ethynyl-2-pyridinamine | |
Source | CAS Common Chemistry | |
URL | https://commonchemistry.cas.org/detail?cas_rn=67346-74-1 | |
Description | CAS Common Chemistry is an open community resource for accessing chemical information. Nearly 500,000 chemical substances from CAS REGISTRY cover areas of community interest, including common and frequently regulated chemicals, and those relevant to high school and undergraduate chemistry classes. This chemical information, curated by our expert scientists, is provided in alignment with our mission as a division of the American Chemical Society. | |
Explanation | The data from CAS Common Chemistry is provided under a CC-BY-NC 4.0 license, unless otherwise stated. | |
Record name | 3-Ethynylpyridin-2-amine | |
Source | EPA DSSTox | |
URL | https://comptox.epa.gov/dashboard/DTXSID70497524 | |
Description | DSSTox provides a high quality public chemistry resource for supporting improved predictive toxicology. | |
Synthesis routes and methods I
Procedure details
Synthesis routes and methods II
Procedure details
Synthesis routes and methods III
Procedure details
Synthesis routes and methods IV
Procedure details
Retrosynthesis Analysis
AI-Powered Synthesis Planning: Our tool employs the Template_relevance Pistachio, Template_relevance Bkms_metabolic, Template_relevance Pistachio_ringbreaker, Template_relevance Reaxys, Template_relevance Reaxys_biocatalysis model, leveraging a vast database of chemical reactions to predict feasible synthetic routes.
One-Step Synthesis Focus: Specifically designed for one-step synthesis, it provides concise and direct routes for your target compounds, streamlining the synthesis process.
Accurate Predictions: Utilizing the extensive PISTACHIO, BKMS_METABOLIC, PISTACHIO_RINGBREAKER, REAXYS, REAXYS_BIOCATALYSIS database, our tool offers high-accuracy predictions, reflecting the latest in chemical research and data.
Strategy Settings
Precursor scoring | Relevance Heuristic |
---|---|
Min. plausibility | 0.01 |
Model | Template_relevance |
Template Set | Pistachio/Bkms_metabolic/Pistachio_ringbreaker/Reaxys/Reaxys_biocatalysis |
Top-N result to add to graph | 6 |
Feasible Synthetic Routes
Disclaimer and Information on In-Vitro Research Products
Please be aware that all articles and product information presented on BenchChem are intended solely for informational purposes. The products available for purchase on BenchChem are specifically designed for in-vitro studies, which are conducted outside of living organisms. In-vitro studies, derived from the Latin term "in glass," involve experiments performed in controlled laboratory settings using cells or tissues. It is important to note that these products are not categorized as medicines or drugs, and they have not received approval from the FDA for the prevention, treatment, or cure of any medical condition, ailment, or disease. We must emphasize that any form of bodily introduction of these products into humans or animals is strictly prohibited by law. It is essential to adhere to these guidelines to ensure compliance with legal and ethical standards in research and experimentation.