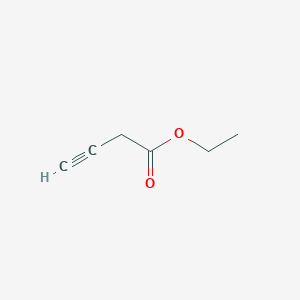
Ethyl 3-butynoate
Overview
Description
Ethyl 3-butynoate is a useful research compound. Its molecular formula is C6H8O2 and its molecular weight is 112.13 g/mol. The purity is usually 95%.
The exact mass of the compound this compound is unknown and the complexity rating of the compound is unknown. The United Nations designated GHS hazard class pictogram is Flammable;Irritant, and the GHS signal word is WarningThe storage condition is unknown. Please store according to label instructions upon receipt of goods.
BenchChem offers high-quality this compound suitable for many research applications. Different packaging options are available to accommodate customers' requirements. Please inquire for more information about this compound including the price, delivery time, and more detailed information at info@benchchem.com.
Mechanism of Action
Target of Action
Ethyl 3-butynoate is a chemical compound used in organic synthesis reactions . It’s important to note that the targets can vary depending on the context of its use, particularly in complex biological systems.
Mode of Action
In general, esters like this compound can participate in reactions such as esterification and transesterification . These reactions involve the interaction of the ester group with other molecules, leading to the formation of new compounds.
Biochemical Pathways
As an ester, it can be involved in various organic synthesis reactions . The products of these reactions can potentially influence various biochemical pathways, depending on the specific context and conditions.
Result of Action
As an ester, it can undergo reactions to form new compounds, which can have various effects depending on their nature and the context in which they are formed .
Action Environment
The action, efficacy, and stability of this compound can be influenced by various environmental factors. For instance, factors such as temperature, pH, and the presence of other chemicals can affect its reactivity and stability . Therefore, these factors should be carefully controlled when using this compound in experimental or industrial applications.
Biochemical Analysis
Biochemical Properties
Ethyl 3-butynoate plays a significant role in biochemical reactions due to its unique structure, which includes an alkyne group. This compound interacts with various enzymes and proteins, influencing their activity. For instance, this compound is known to undergo codimerization with alkenes to form 1,3-dienes, a reaction catalyzed by rhodium (I)/H8-BINAP complex . Additionally, it acts as a methanogenesis inhibitor, affecting microbial biomass production . These interactions highlight the compound’s versatility in biochemical processes.
Cellular Effects
This compound has notable effects on cellular processes. It influences cell function by interacting with cellular enzymes and proteins, thereby affecting cell signaling pathways, gene expression, and cellular metabolism. For example, this compound has been shown to inhibit methanogenesis, which can impact microbial cell metabolism and biomass production . This inhibition can lead to changes in cellular energy production and overall cell function.
Molecular Mechanism
The molecular mechanism of this compound involves its interaction with various biomolecules. At the molecular level, this compound exerts its effects through binding interactions with enzymes and proteins. For instance, it can inhibit enzyme activity by binding to the active site or altering the enzyme’s conformation. This inhibition can lead to changes in gene expression and cellular metabolism, as seen in its role as a methanogenesis inhibitor .
Temporal Effects in Laboratory Settings
In laboratory settings, the effects of this compound can change over time. The compound’s stability and degradation are crucial factors in its long-term effects on cellular function. This compound is relatively stable under standard laboratory conditions, but its degradation products can influence cellular processes. Long-term studies have shown that this compound can have sustained effects on microbial biomass production and enzyme activity .
Dosage Effects in Animal Models
The effects of this compound vary with different dosages in animal models. At low doses, the compound may have minimal impact on cellular function, while higher doses can lead to significant changes in enzyme activity and cellular metabolism. Toxic or adverse effects may occur at high doses, highlighting the importance of dosage control in experimental settings .
Metabolic Pathways
This compound is involved in various metabolic pathways, interacting with enzymes and cofactors. It can influence metabolic flux and metabolite levels, particularly in pathways related to energy production and biomass synthesis. For example, its role as a methanogenesis inhibitor affects the metabolic pathways of microbial cells, leading to changes in energy production and metabolite levels .
Transport and Distribution
Within cells and tissues, this compound is transported and distributed through interactions with transporters and binding proteins. These interactions can affect the compound’s localization and accumulation in specific cellular compartments. This compound’s transport and distribution are crucial for its biochemical effects, as they determine the compound’s availability to interact with enzymes and proteins .
Subcellular Localization
This compound’s subcellular localization is influenced by targeting signals and post-translational modifications. These factors direct the compound to specific compartments or organelles, where it can exert its biochemical effects. For instance, this compound may localize to the mitochondria or endoplasmic reticulum, affecting cellular metabolism and enzyme activity .
Properties
IUPAC Name |
ethyl but-3-ynoate | |
---|---|---|
Source | PubChem | |
URL | https://pubchem.ncbi.nlm.nih.gov | |
Description | Data deposited in or computed by PubChem | |
InChI |
InChI=1S/C6H8O2/c1-3-5-6(7)8-4-2/h1H,4-5H2,2H3 | |
Source | PubChem | |
URL | https://pubchem.ncbi.nlm.nih.gov | |
Description | Data deposited in or computed by PubChem | |
InChI Key |
YUIKUBSCWKOQKS-UHFFFAOYSA-N | |
Source | PubChem | |
URL | https://pubchem.ncbi.nlm.nih.gov | |
Description | Data deposited in or computed by PubChem | |
Canonical SMILES |
CCOC(=O)CC#C | |
Source | PubChem | |
URL | https://pubchem.ncbi.nlm.nih.gov | |
Description | Data deposited in or computed by PubChem | |
Molecular Formula |
C6H8O2 | |
Source | PubChem | |
URL | https://pubchem.ncbi.nlm.nih.gov | |
Description | Data deposited in or computed by PubChem | |
DSSTOX Substance ID |
DTXSID50466415 | |
Record name | ETHYL 3-BUTYNOATE | |
Source | EPA DSSTox | |
URL | https://comptox.epa.gov/dashboard/DTXSID50466415 | |
Description | DSSTox provides a high quality public chemistry resource for supporting improved predictive toxicology. | |
Molecular Weight |
112.13 g/mol | |
Source | PubChem | |
URL | https://pubchem.ncbi.nlm.nih.gov | |
Description | Data deposited in or computed by PubChem | |
CAS No. |
53841-07-9 | |
Record name | ETHYL 3-BUTYNOATE | |
Source | EPA DSSTox | |
URL | https://comptox.epa.gov/dashboard/DTXSID50466415 | |
Description | DSSTox provides a high quality public chemistry resource for supporting improved predictive toxicology. | |
Record name | ethyl but-3-ynoate | |
Source | European Chemicals Agency (ECHA) | |
URL | https://echa.europa.eu/information-on-chemicals | |
Description | The European Chemicals Agency (ECHA) is an agency of the European Union which is the driving force among regulatory authorities in implementing the EU's groundbreaking chemicals legislation for the benefit of human health and the environment as well as for innovation and competitiveness. | |
Explanation | Use of the information, documents and data from the ECHA website is subject to the terms and conditions of this Legal Notice, and subject to other binding limitations provided for under applicable law, the information, documents and data made available on the ECHA website may be reproduced, distributed and/or used, totally or in part, for non-commercial purposes provided that ECHA is acknowledged as the source: "Source: European Chemicals Agency, http://echa.europa.eu/". Such acknowledgement must be included in each copy of the material. ECHA permits and encourages organisations and individuals to create links to the ECHA website under the following cumulative conditions: Links can only be made to webpages that provide a link to the Legal Notice page. | |
Retrosynthesis Analysis
AI-Powered Synthesis Planning: Our tool employs the Template_relevance Pistachio, Template_relevance Bkms_metabolic, Template_relevance Pistachio_ringbreaker, Template_relevance Reaxys, Template_relevance Reaxys_biocatalysis model, leveraging a vast database of chemical reactions to predict feasible synthetic routes.
One-Step Synthesis Focus: Specifically designed for one-step synthesis, it provides concise and direct routes for your target compounds, streamlining the synthesis process.
Accurate Predictions: Utilizing the extensive PISTACHIO, BKMS_METABOLIC, PISTACHIO_RINGBREAKER, REAXYS, REAXYS_BIOCATALYSIS database, our tool offers high-accuracy predictions, reflecting the latest in chemical research and data.
Strategy Settings
Precursor scoring | Relevance Heuristic |
---|---|
Min. plausibility | 0.01 |
Model | Template_relevance |
Template Set | Pistachio/Bkms_metabolic/Pistachio_ringbreaker/Reaxys/Reaxys_biocatalysis |
Top-N result to add to graph | 6 |
Feasible Synthetic Routes
Q1: What is the main chemical reaction studied in the paper involving Ethyl 3-butynoate?
A1: The paper investigates the electrophilic addition reactions of hydrogen halides (HCl, HBr, HI) and their deuterated counterparts (DCl, DBr, DI) to this compound []. This research focuses on understanding the regioselectivity and stereoselectivity of these additions, which are fundamental concepts in organic chemistry.
Q2: Why is understanding the regioselectivity and stereoselectivity of these reactions important?
A2: Regioselectivity refers to the preference of a chemical reaction to occur at a specific site when multiple possibilities exist within a molecule. Stereoselectivity refers to the preferential formation of one stereoisomer over another in a chemical reaction. Understanding these selectivities in the context of this compound helps predict the major products formed upon reaction with hydrogen halides. This knowledge is crucial for synthetic chemists designing reaction pathways and predicting product outcomes [].
Disclaimer and Information on In-Vitro Research Products
Please be aware that all articles and product information presented on BenchChem are intended solely for informational purposes. The products available for purchase on BenchChem are specifically designed for in-vitro studies, which are conducted outside of living organisms. In-vitro studies, derived from the Latin term "in glass," involve experiments performed in controlled laboratory settings using cells or tissues. It is important to note that these products are not categorized as medicines or drugs, and they have not received approval from the FDA for the prevention, treatment, or cure of any medical condition, ailment, or disease. We must emphasize that any form of bodily introduction of these products into humans or animals is strictly prohibited by law. It is essential to adhere to these guidelines to ensure compliance with legal and ethical standards in research and experimentation.