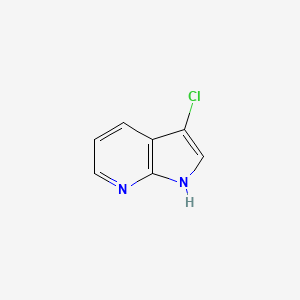
3-Chloro-7-azaindole
Overview
Description
3-Chloro-7-azaindole is a heterocyclic compound that belongs to the azaindole family. This compound is characterized by the presence of a chlorine atom at the third position and a nitrogen atom at the seventh position of the indole ring. Azaindoles are known for their significant biological activities and have been widely studied for their potential therapeutic applications .
Mechanism of Action
Target of Action
3-Chloro-7-azaindole, like other azaindole derivatives, is primarily targeted towards protein kinases . These protein kinases play a key role in the growth, proliferation, differentiation, migration, and apoptosis of cells . The compound has shown potential to be developed into a multi-targeted kinase inhibitor .
Mode of Action
The compound interacts with its targets, the protein kinases, by binding to them. The binding efficacy of this compound with protein kinases was analyzed through molecular docking studies . The smaller the lowest binding energy data between the compound and each target, the better the anticancer activity it showed .
Biochemical Pathways
The biochemical pathways affected by this compound are those regulated by the protein kinases it targets. Protein kinases play a key role in various cellular processes, including cell growth, proliferation, differentiation, migration, and apoptosis . By inhibiting these kinases, this compound can disrupt these processes, particularly in cancer cells where these pathways are often dysregulated .
Pharmacokinetics
Azaindoles in general have been recognized as privileged structures in biological process modulation, in medicinal chemistry, and drug discovery programs . They have the potential to modulate and finely tune target binding and ADME-tox properties .
Result of Action
The primary result of this compound’s action is its antiproliferative activity. It has been tested against various cell lines and has shown significant results . For instance, it has shown effective inhibitory concentration (IC50) on HeLa cells, MCF-7, and MDA MB-231 cells . The compound effectively inhibited DDX3 in a dose-dependent manner .
Biochemical Analysis
Biochemical Properties
3-Chloro-7-azaindole plays a crucial role in biochemical reactions, particularly as an inhibitor of protein kinases. Protein kinases are enzymes that catalyze the transfer of phosphate groups from adenosine triphosphate to specific substrates, a process essential for regulating cellular activities. This compound has been shown to inhibit cyclin-dependent kinase 9 (CDK9) and Haspin kinase, both of which are involved in cell cycle regulation and gene expression . The interaction between this compound and these kinases involves binding to the adenosine triphosphate-binding site, thereby preventing the phosphorylation of target proteins .
Cellular Effects
The effects of this compound on various cell types and cellular processes are profound. By inhibiting CDK9, this compound disrupts the transcriptional elongation of genes, leading to altered gene expression profiles . This inhibition can result in the suppression of oncogenes, making this compound a potential anticancer agent. Additionally, the inhibition of Haspin kinase by this compound affects chromosomal alignment during mitosis, further influencing cell division and proliferation .
Molecular Mechanism
At the molecular level, this compound exerts its effects through specific binding interactions with protein kinases. The compound binds to the adenosine triphosphate-binding pocket of CDK9 and Haspin kinase, leading to competitive inhibition . This binding prevents the transfer of phosphate groups to substrate proteins, thereby inhibiting kinase activity. The resulting decrease in phosphorylation events alters downstream signaling pathways and gene expression, contributing to the compound’s biological effects .
Temporal Effects in Laboratory Settings
In laboratory settings, the effects of this compound have been observed to change over time. The compound exhibits stability under standard storage conditions, but its activity may decrease upon prolonged exposure to light and air . In vitro studies have shown that the inhibitory effects of this compound on CDK9 and Haspin kinase are sustained over several hours, with gradual degradation observed over extended periods . Long-term exposure to this compound in cell cultures can lead to persistent changes in gene expression and cellular function .
Dosage Effects in Animal Models
The effects of this compound vary with different dosages in animal models. At low doses, the compound effectively inhibits target kinases without causing significant toxicity . Higher doses of this compound can lead to adverse effects, including hepatotoxicity and nephrotoxicity . Threshold effects have been observed, where a certain dosage is required to achieve therapeutic benefits without inducing toxicity . These findings highlight the importance of dose optimization in the potential therapeutic use of this compound.
Metabolic Pathways
This compound is involved in metabolic pathways that include its biotransformation by liver enzymes. Cytochrome P450 enzymes play a key role in the metabolism of this compound, leading to the formation of various metabolites . These metabolites can further interact with cellular targets, contributing to the overall biological effects of the compound. The metabolic flux and levels of metabolites are influenced by the activity of these enzymes, which can vary among individuals .
Transport and Distribution
Within cells and tissues, this compound is transported and distributed through passive diffusion and active transport mechanisms . The compound can interact with transporters and binding proteins that facilitate its movement across cellular membranes . Once inside the cell, this compound can accumulate in specific compartments, influencing its localization and activity . The distribution of this compound within tissues is also affected by factors such as blood flow and tissue permeability .
Subcellular Localization
The subcellular localization of this compound is critical for its activity and function. The compound can be directed to specific cellular compartments through targeting signals and post-translational modifications . For example, this compound may localize to the nucleus, where it interacts with nuclear kinases and influences gene expression . The localization of this compound to other organelles, such as mitochondria, can also impact its biological effects and therapeutic potential .
Preparation Methods
Synthetic Routes and Reaction Conditions: The synthesis of 3-Chloro-7-azaindole can be achieved through various methods. One common approach involves the Suzuki coupling reaction, where halogenated 7-azaindole is reacted with boronic acids under palladium-catalyzed conditions . Another method includes the microwave-assisted synthesis, which involves the reaction of o-haloaromatic amine with terminal alkynes under microwave irradiation .
Industrial Production Methods: Industrial production of this compound typically involves large-scale synthesis using optimized reaction conditions to ensure high yield and purity. The use of continuous flow reactors and advanced catalytic systems can enhance the efficiency of the production process .
Chemical Reactions Analysis
Types of Reactions: 3-Chloro-7-azaindole undergoes various chemical reactions, including:
Substitution Reactions: The chlorine atom can be substituted with other functional groups using nucleophilic substitution reactions.
Oxidation and Reduction Reactions: The compound can undergo oxidation to form corresponding oxides or reduction to form reduced derivatives.
Cross-Coupling Reactions: Palladium-catalyzed cross-coupling reactions, such as Suzuki and Heck reactions, are commonly used to functionalize the azaindole ring.
Common Reagents and Conditions:
Palladium Catalysts: Used in cross-coupling reactions.
Nucleophiles: Employed in substitution reactions.
Oxidizing and Reducing Agents: Utilized in oxidation and reduction reactions.
Major Products Formed: The major products formed from these reactions include various substituted azaindole derivatives, which can be further explored for their biological activities .
Scientific Research Applications
Chemistry: Used as a building block for the synthesis of complex organic molecules.
Comparison with Similar Compounds
7-Azaindole: Lacks the chlorine atom at the third position but shares the same core structure.
4-Chloro-7-azaindole: Similar structure with the chlorine atom at the fourth position.
5-Chloro-7-azaindole: Chlorine atom at the fifth position.
Uniqueness: 3-Chloro-7-azaindole is unique due to the specific positioning of the chlorine atom, which can influence its reactivity and biological activity. The presence of the chlorine atom at the third position can enhance its binding affinity to certain molecular targets, making it a valuable compound for drug discovery and development .
Properties
IUPAC Name |
3-chloro-1H-pyrrolo[2,3-b]pyridine | |
---|---|---|
Source | PubChem | |
URL | https://pubchem.ncbi.nlm.nih.gov | |
Description | Data deposited in or computed by PubChem | |
InChI |
InChI=1S/C7H5ClN2/c8-6-4-10-7-5(6)2-1-3-9-7/h1-4H,(H,9,10) | |
Source | PubChem | |
URL | https://pubchem.ncbi.nlm.nih.gov | |
Description | Data deposited in or computed by PubChem | |
InChI Key |
PKFDDUMFTQHVFY-UHFFFAOYSA-N | |
Source | PubChem | |
URL | https://pubchem.ncbi.nlm.nih.gov | |
Description | Data deposited in or computed by PubChem | |
Canonical SMILES |
C1=CC2=C(NC=C2Cl)N=C1 | |
Source | PubChem | |
URL | https://pubchem.ncbi.nlm.nih.gov | |
Description | Data deposited in or computed by PubChem | |
Molecular Formula |
C7H5ClN2 | |
Source | PubChem | |
URL | https://pubchem.ncbi.nlm.nih.gov | |
Description | Data deposited in or computed by PubChem | |
DSSTOX Substance ID |
DTXSID50470698 | |
Record name | 3-Chloro-7-azaindole | |
Source | EPA DSSTox | |
URL | https://comptox.epa.gov/dashboard/DTXSID50470698 | |
Description | DSSTox provides a high quality public chemistry resource for supporting improved predictive toxicology. | |
Molecular Weight |
152.58 g/mol | |
Source | PubChem | |
URL | https://pubchem.ncbi.nlm.nih.gov | |
Description | Data deposited in or computed by PubChem | |
CAS No. |
80235-01-4 | |
Record name | 3-Chloro-7-azaindole | |
Source | EPA DSSTox | |
URL | https://comptox.epa.gov/dashboard/DTXSID50470698 | |
Description | DSSTox provides a high quality public chemistry resource for supporting improved predictive toxicology. | |
Record name | 3-Chloro-7-azaindole | |
Source | European Chemicals Agency (ECHA) | |
URL | https://echa.europa.eu/information-on-chemicals | |
Description | The European Chemicals Agency (ECHA) is an agency of the European Union which is the driving force among regulatory authorities in implementing the EU's groundbreaking chemicals legislation for the benefit of human health and the environment as well as for innovation and competitiveness. | |
Explanation | Use of the information, documents and data from the ECHA website is subject to the terms and conditions of this Legal Notice, and subject to other binding limitations provided for under applicable law, the information, documents and data made available on the ECHA website may be reproduced, distributed and/or used, totally or in part, for non-commercial purposes provided that ECHA is acknowledged as the source: "Source: European Chemicals Agency, http://echa.europa.eu/". Such acknowledgement must be included in each copy of the material. ECHA permits and encourages organisations and individuals to create links to the ECHA website under the following cumulative conditions: Links can only be made to webpages that provide a link to the Legal Notice page. | |
Retrosynthesis Analysis
AI-Powered Synthesis Planning: Our tool employs the Template_relevance Pistachio, Template_relevance Bkms_metabolic, Template_relevance Pistachio_ringbreaker, Template_relevance Reaxys, Template_relevance Reaxys_biocatalysis model, leveraging a vast database of chemical reactions to predict feasible synthetic routes.
One-Step Synthesis Focus: Specifically designed for one-step synthesis, it provides concise and direct routes for your target compounds, streamlining the synthesis process.
Accurate Predictions: Utilizing the extensive PISTACHIO, BKMS_METABOLIC, PISTACHIO_RINGBREAKER, REAXYS, REAXYS_BIOCATALYSIS database, our tool offers high-accuracy predictions, reflecting the latest in chemical research and data.
Strategy Settings
Precursor scoring | Relevance Heuristic |
---|---|
Min. plausibility | 0.01 |
Model | Template_relevance |
Template Set | Pistachio/Bkms_metabolic/Pistachio_ringbreaker/Reaxys/Reaxys_biocatalysis |
Top-N result to add to graph | 6 |
Feasible Synthetic Routes
Q1: What makes 3-Chloro-7-azaindole interesting for anticancer research?
A1: this compound belongs to the 7-azaindole family, which demonstrates notable activity as ligands in platinum-based anticancer complexes [, ]. These complexes, structurally similar to cisplatin, hold promise for improved therapeutic outcomes.
Q2: How does the structure of this compound contribute to its activity in platinum complexes?
A2: While the provided abstracts don't delve into the specific mechanism, research suggests that 7-azaindole derivatives, including this compound, can coordinate to platinum through their nitrogen atoms [, ]. This interaction likely influences the complex's interaction with DNA, potentially leading to enhanced antitumor activity. Further studies are needed to confirm the exact mechanism of action.
Q3: Are there any studies investigating the toxicity of this compound-containing platinum complexes?
A3: Yes, research has explored the toxic effects of cis-dichloridoplatinum(II) complexes containing this compound and similar 7-azaindole halogen derivatives in tumor cells []. This research highlights the importance of understanding the safety profile of these complexes for potential therapeutic applications.
Q4: What are the challenges in formulating this compound-containing complexes for drug delivery?
A4: One study successfully utilized 4-aminobenzoic acid-coated maghemite nanoparticles to deliver a highly cytotoxic cisplatin-like complex incorporating this compound []. This suggests the potential of nanoformulations in overcoming challenges like solubility and targeted delivery, paving the way for more effective cancer therapies.
Q5: Are there analytical techniques available to study this compound?
A5: Various techniques have been employed to characterize this compound. X-ray crystallography has elucidated its structure, revealing dual N-H⋯N hydrogen bonds in its dimeric form []. Additionally, vibrational spectroscopy, potentially including infrared and Raman spectroscopy, has been used to study its vibrational modes [].
Disclaimer and Information on In-Vitro Research Products
Please be aware that all articles and product information presented on BenchChem are intended solely for informational purposes. The products available for purchase on BenchChem are specifically designed for in-vitro studies, which are conducted outside of living organisms. In-vitro studies, derived from the Latin term "in glass," involve experiments performed in controlled laboratory settings using cells or tissues. It is important to note that these products are not categorized as medicines or drugs, and they have not received approval from the FDA for the prevention, treatment, or cure of any medical condition, ailment, or disease. We must emphasize that any form of bodily introduction of these products into humans or animals is strictly prohibited by law. It is essential to adhere to these guidelines to ensure compliance with legal and ethical standards in research and experimentation.