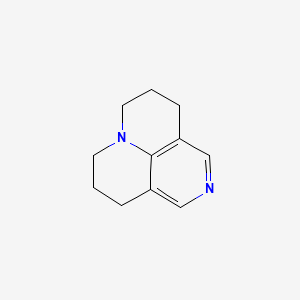
9-Azajulolidine
Overview
Description
Mechanism of Action
Target of Action
9-Azajulolidine is primarily used as an electron-rich ligand in chemical reactions . It is particularly effective in post-Ullmann-type reactions, which involve the formation of C(aryl)–heteroatom (N, O, S) bonds .
Mode of Action
This compound interacts with its targets by acting as a catalyst . It enhances the reactivity of certain reactions, such as the acylation of sterically hindered alcohols . This is attributed to the high stability of their intermediate N-acylpyridinium ions, a consequence of the relatively high electron densities on their pyridine rings .
Biochemical Pathways
This compound is involved in the acylation and silylation of alcohols and amines, trans-esterification, amide formation, Baylis–Hillman reactions, and nucleophilic substitutions . It is also used in the synthesis of phosphoramidites, which are essential for the production of oligonucleotides .
Result of Action
The use of this compound in reactions results in dramatically enhanced reaction rates . For instance, it has been demonstrated to display remarkably enhanced catalytic activities toward the acylation of sterically hindered alcohols .
Action Environment
The action of this compound can be influenced by various environmental factors. Additionally, the compound’s reactivity can be affected by the presence of other compounds in the reaction environment .
Biochemical Analysis
Biochemical Properties
9-Azajulolidine plays a significant role in biochemical reactions, particularly in catalytic processes. It acts as an effective electron-rich ligand for post-Ullmann-type reactions, which involve the formation of carbon-heteroatom bonds such as carbon-nitrogen, carbon-oxygen, and carbon-sulfur bonds . The compound’s high electron density and conformational restriction of the amino group contribute to its enhanced catalytic activity.
In these reactions, this compound interacts with various enzymes and proteins, including copper salts and nitrogen-containing heteroarenes like pyridine, bipyridine, terpyridine, and 1,10-phenanthroline . These interactions facilitate the formation of stable intermediate complexes, thereby accelerating the reaction rates and improving the overall efficiency of the catalytic process.
Cellular Effects
The effects of this compound on cellular processes are multifaceted. It influences cell function by modulating cell signaling pathways, gene expression, and cellular metabolism. The compound’s ability to act as a Lewis base and nucleophilic catalyst enables it to participate in acylation reactions, which are crucial for the modification of proteins and other biomolecules .
This compound has been shown to enhance the catalytic activity of acylation reactions, leading to increased stability of intermediate N-acylpyridinium ions . This stabilization effect can impact various cellular processes, including protein synthesis, signal transduction, and metabolic regulation.
Molecular Mechanism
At the molecular level, this compound exerts its effects through several mechanisms. It acts as a Lewis base, facilitating the formation of stable complexes with electrophilic species. This interaction enhances the reactivity of the compound in catalytic processes, particularly in the acylation of sterically hindered alcohols .
The compound’s high electron density and conformational restriction of the amino group contribute to its ability to stabilize intermediate complexes. This stabilization effect is primarily due to the inductive electron-donating effect of the alkyl groups and the conformational restriction of the amino group . These properties enable this compound to act as an effective catalyst in various biochemical reactions.
Temporal Effects in Laboratory Settings
In laboratory settings, the effects of this compound can vary over time. The compound is relatively stable under ambient conditions, but its catalytic activity may decrease over extended periods due to potential degradation or changes in its chemical structure . Long-term studies have shown that this compound maintains its catalytic efficiency for several hours, but prolonged exposure to air or moisture can lead to a gradual decline in its activity .
Dosage Effects in Animal Models
The effects of this compound in animal models are dose-dependent. At low doses, the compound exhibits minimal toxicity and can effectively catalyze biochemical reactions without adverse effects . At higher doses, this compound may induce toxic effects, including cellular damage and disruption of metabolic processes . Threshold effects have been observed, where the compound’s catalytic activity reaches a peak at an optimal dosage, beyond which its efficacy diminishes, and toxicity increases .
Metabolic Pathways
This compound is involved in several metabolic pathways, primarily those related to acylation and nucleophilic substitution reactions. The compound interacts with enzymes such as acyltransferases and nucleophilic catalysts, facilitating the transfer of acyl groups to target molecules . This interaction can influence metabolic flux and alter the levels of various metabolites within the cell .
Transport and Distribution
Within cells and tissues, this compound is transported and distributed through interactions with specific transporters and binding proteins. The compound’s high electron density and conformational restriction enable it to bind effectively to these transporters, facilitating its movement across cellular membranes . This transport mechanism ensures that this compound reaches its target sites within the cell, where it can exert its catalytic effects.
Subcellular Localization
The subcellular localization of this compound is influenced by its chemical properties and interactions with cellular components. The compound is primarily localized in the cytoplasm, where it participates in various biochemical reactions . Targeting signals and post-translational modifications may direct this compound to specific compartments or organelles, enhancing its catalytic activity and functional specificity .
Preparation Methods
The synthesis of 9-azajulolidine can be achieved through various methods. One efficient synthetic route involves the use of readily available reagents and its application as an electron-rich ligand for post-Ullmann-type reactions . The reaction conditions typically involve the use of copper salts and nitrogen-containing heteroarenes as co-catalysts. The process is known for its high reaction rates and efficiency .
Chemical Reactions Analysis
9-Azajulolidine undergoes several types of chemical reactions, including:
Oxidation and Reduction: It can participate in redox reactions, where it can either donate or accept electrons.
Substitution Reactions: It is involved in nucleophilic substitutions, particularly in the acylation and silylation of alcohols and amines.
Coupling Reactions: It is used as an auxiliary ligand in post-Ullmann reactions for the formation of C(aryl)–N, C(aryl)–O, and C(aryl)–S bonds.
Common reagents used in these reactions include copper iodide (CuI) as a catalyst, sodium tert-butoxide (NaOtBu) as a base, and various aryl iodides . The major products formed from these reactions are typically triarylamines, which are important in organic optoelectronic devices .
Scientific Research Applications
9-Azajulolidine has a wide range of applications in scientific research:
Comparison with Similar Compounds
9-Azajulolidine is often compared with other similar compounds such as:
4-Dimethylaminopyridine (DMAP): Known for its catalytic activity in acylation reactions, but this compound exhibits higher catalytic activity due to its electron-rich nature.
4-Pyrolidinopyridine (PPY): Another catalyst for nucleophilic substitutions, but this compound is more reactive due to its conformationally restricted structure.
1,1,7,7-Tetramethyl-9-azajulolidine (TMAJ): A highly active derivative of this compound, showing even higher catalytic activity in certain reactions.
Properties
IUPAC Name |
1,7-diazatricyclo[7.3.1.05,13]trideca-5,7,9(13)-triene | |
---|---|---|
Source | PubChem | |
URL | https://pubchem.ncbi.nlm.nih.gov | |
Description | Data deposited in or computed by PubChem | |
InChI |
InChI=1S/C11H14N2/c1-3-9-7-12-8-10-4-2-6-13(5-1)11(9)10/h7-8H,1-6H2 | |
Source | PubChem | |
URL | https://pubchem.ncbi.nlm.nih.gov | |
Description | Data deposited in or computed by PubChem | |
InChI Key |
VARHFUNXFXTFII-UHFFFAOYSA-N | |
Source | PubChem | |
URL | https://pubchem.ncbi.nlm.nih.gov | |
Description | Data deposited in or computed by PubChem | |
Canonical SMILES |
C1CC2=CN=CC3=C2N(C1)CCC3 | |
Source | PubChem | |
URL | https://pubchem.ncbi.nlm.nih.gov | |
Description | Data deposited in or computed by PubChem | |
Molecular Formula |
C11H14N2 | |
Source | PubChem | |
URL | https://pubchem.ncbi.nlm.nih.gov | |
Description | Data deposited in or computed by PubChem | |
DSSTOX Substance ID |
DTXSID80464020 | |
Record name | 9-Azajulolidine | |
Source | EPA DSSTox | |
URL | https://comptox.epa.gov/dashboard/DTXSID80464020 | |
Description | DSSTox provides a high quality public chemistry resource for supporting improved predictive toxicology. | |
Molecular Weight |
174.24 g/mol | |
Source | PubChem | |
URL | https://pubchem.ncbi.nlm.nih.gov | |
Description | Data deposited in or computed by PubChem | |
CAS No. |
6052-72-8 | |
Record name | 9-Azajulolidine | |
Source | EPA DSSTox | |
URL | https://comptox.epa.gov/dashboard/DTXSID80464020 | |
Description | DSSTox provides a high quality public chemistry resource for supporting improved predictive toxicology. | |
Retrosynthesis Analysis
AI-Powered Synthesis Planning: Our tool employs the Template_relevance Pistachio, Template_relevance Bkms_metabolic, Template_relevance Pistachio_ringbreaker, Template_relevance Reaxys, Template_relevance Reaxys_biocatalysis model, leveraging a vast database of chemical reactions to predict feasible synthetic routes.
One-Step Synthesis Focus: Specifically designed for one-step synthesis, it provides concise and direct routes for your target compounds, streamlining the synthesis process.
Accurate Predictions: Utilizing the extensive PISTACHIO, BKMS_METABOLIC, PISTACHIO_RINGBREAKER, REAXYS, REAXYS_BIOCATALYSIS database, our tool offers high-accuracy predictions, reflecting the latest in chemical research and data.
Strategy Settings
Precursor scoring | Relevance Heuristic |
---|---|
Min. plausibility | 0.01 |
Model | Template_relevance |
Template Set | Pistachio/Bkms_metabolic/Pistachio_ringbreaker/Reaxys/Reaxys_biocatalysis |
Top-N result to add to graph | 6 |
Feasible Synthetic Routes
Disclaimer and Information on In-Vitro Research Products
Please be aware that all articles and product information presented on BenchChem are intended solely for informational purposes. The products available for purchase on BenchChem are specifically designed for in-vitro studies, which are conducted outside of living organisms. In-vitro studies, derived from the Latin term "in glass," involve experiments performed in controlled laboratory settings using cells or tissues. It is important to note that these products are not categorized as medicines or drugs, and they have not received approval from the FDA for the prevention, treatment, or cure of any medical condition, ailment, or disease. We must emphasize that any form of bodily introduction of these products into humans or animals is strictly prohibited by law. It is essential to adhere to these guidelines to ensure compliance with legal and ethical standards in research and experimentation.