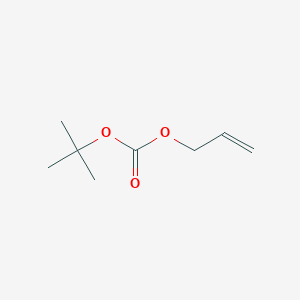
Allyl tert-butyl carbonate
Overview
Description
Allyl tert-butyl carbonate is an organic compound with the molecular formula C8H14O3. It is a colorless liquid that is used primarily as a reagent in organic synthesis. The compound is known for its utility in various chemical reactions, particularly in the formation of carbon-carbon and carbon-oxygen bonds.
Preparation Methods
Synthetic Routes and Reaction Conditions: Allyl tert-butyl carbonate can be synthesized through the reaction of allyl alcohol with tert-butyl chloroformate in the presence of a base such as pyridine. The reaction typically proceeds under mild conditions and yields the desired carbonate ester.
Industrial Production Methods: In an industrial setting, the synthesis of this compound may involve the use of continuous flow reactors to optimize the reaction conditions and improve yield. The process generally includes the careful control of temperature and pressure to ensure the efficient production of the compound.
Types of Reactions:
Oxidation: this compound can undergo oxidation reactions to form various oxidized products.
Reduction: The compound can be reduced to form allyl alcohol and tert-butyl alcohol.
Substitution: this compound can participate in substitution reactions, where the allyl group is replaced by other functional groups.
Common Reagents and Conditions:
Oxidation: Common oxidizing agents include potassium permanganate and chromium trioxide.
Reduction: Reducing agents such as lithium aluminum hydride and sodium borohydride are typically used.
Substitution: Nucleophiles such as amines and thiols can be used in substitution reactions.
Major Products Formed:
Oxidation: Products may include allyl aldehyde and tert-butyl formate.
Reduction: Products include allyl alcohol and tert-butyl alcohol.
Substitution: Products vary depending on the nucleophile used, but may include allyl amines and allyl thiols.
Scientific Research Applications
Allyl tert-butyl carbonate is widely used in scientific research due to its versatility in organic synthesis. It is used in the preparation of various pharmaceuticals, agrochemicals, and fine chemicals. The compound is also employed in the synthesis of complex molecules in medicinal chemistry and material science.
Mechanism of Action
The mechanism of action of allyl tert-butyl carbonate involves the formation of reactive intermediates such as allyl cations and tert-butyl cations. These intermediates can undergo various chemical transformations, leading to the formation of new carbon-carbon and carbon-oxygen bonds. The molecular targets and pathways involved depend on the specific reaction conditions and reagents used.
Comparison with Similar Compounds
- Allyl methyl carbonate
- Allyl ethyl carbonate
- Allyl isopropyl carbonate
Comparison: Allyl tert-butyl carbonate is unique due to the presence of the bulky tert-butyl group, which can influence the reactivity and selectivity of the compound in chemical reactions. Compared to other allyl carbonates, this compound may exhibit different reaction rates and product distributions due to steric and electronic effects.
Properties
IUPAC Name |
tert-butyl prop-2-enyl carbonate | |
---|---|---|
Source | PubChem | |
URL | https://pubchem.ncbi.nlm.nih.gov | |
Description | Data deposited in or computed by PubChem | |
InChI |
InChI=1S/C8H14O3/c1-5-6-10-7(9)11-8(2,3)4/h5H,1,6H2,2-4H3 | |
Source | PubChem | |
URL | https://pubchem.ncbi.nlm.nih.gov | |
Description | Data deposited in or computed by PubChem | |
InChI Key |
SWQDRYKDDGFPLL-UHFFFAOYSA-N | |
Source | PubChem | |
URL | https://pubchem.ncbi.nlm.nih.gov | |
Description | Data deposited in or computed by PubChem | |
Canonical SMILES |
CC(C)(C)OC(=O)OCC=C | |
Source | PubChem | |
URL | https://pubchem.ncbi.nlm.nih.gov | |
Description | Data deposited in or computed by PubChem | |
Molecular Formula |
C8H14O3 | |
Source | PubChem | |
URL | https://pubchem.ncbi.nlm.nih.gov | |
Description | Data deposited in or computed by PubChem | |
DSSTOX Substance ID |
DTXSID30458257 | |
Record name | ALLYL TERT-BUTYL CARBONATE | |
Source | EPA DSSTox | |
URL | https://comptox.epa.gov/dashboard/DTXSID30458257 | |
Description | DSSTox provides a high quality public chemistry resource for supporting improved predictive toxicology. | |
Molecular Weight |
158.19 g/mol | |
Source | PubChem | |
URL | https://pubchem.ncbi.nlm.nih.gov | |
Description | Data deposited in or computed by PubChem | |
CAS No. |
70122-89-3 | |
Record name | ALLYL TERT-BUTYL CARBONATE | |
Source | EPA DSSTox | |
URL | https://comptox.epa.gov/dashboard/DTXSID30458257 | |
Description | DSSTox provides a high quality public chemistry resource for supporting improved predictive toxicology. | |
Retrosynthesis Analysis
AI-Powered Synthesis Planning: Our tool employs the Template_relevance Pistachio, Template_relevance Bkms_metabolic, Template_relevance Pistachio_ringbreaker, Template_relevance Reaxys, Template_relevance Reaxys_biocatalysis model, leveraging a vast database of chemical reactions to predict feasible synthetic routes.
One-Step Synthesis Focus: Specifically designed for one-step synthesis, it provides concise and direct routes for your target compounds, streamlining the synthesis process.
Accurate Predictions: Utilizing the extensive PISTACHIO, BKMS_METABOLIC, PISTACHIO_RINGBREAKER, REAXYS, REAXYS_BIOCATALYSIS database, our tool offers high-accuracy predictions, reflecting the latest in chemical research and data.
Strategy Settings
Precursor scoring | Relevance Heuristic |
---|---|
Min. plausibility | 0.01 |
Model | Template_relevance |
Template Set | Pistachio/Bkms_metabolic/Pistachio_ringbreaker/Reaxys/Reaxys_biocatalysis |
Top-N result to add to graph | 6 |
Feasible Synthetic Routes
Disclaimer and Information on In-Vitro Research Products
Please be aware that all articles and product information presented on BenchChem are intended solely for informational purposes. The products available for purchase on BenchChem are specifically designed for in-vitro studies, which are conducted outside of living organisms. In-vitro studies, derived from the Latin term "in glass," involve experiments performed in controlled laboratory settings using cells or tissues. It is important to note that these products are not categorized as medicines or drugs, and they have not received approval from the FDA for the prevention, treatment, or cure of any medical condition, ailment, or disease. We must emphasize that any form of bodily introduction of these products into humans or animals is strictly prohibited by law. It is essential to adhere to these guidelines to ensure compliance with legal and ethical standards in research and experimentation.