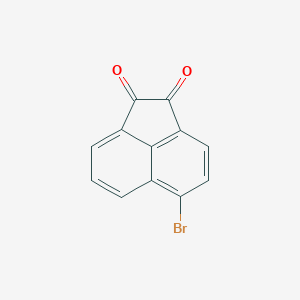
1,2-Acenaphthylenedione, 5-bromo-
Overview
Description
5-Bromoacenaphthene (CAS: 19190-91-1) is a brominated derivative of acenaphthene, a polycyclic aromatic hydrocarbon (PAH). Its structure consists of a naphthalene core fused with a cyclopentane ring, with a bromine substituent at the 5-position. The compound is characterized by the molecular formula C₁₂H₉Br and a melting point of 52°C . Its synthesis typically involves Friedel-Crafts acylation or bromination reactions, as exemplified by the use of bromoacetyl bromide and aluminum chloride in dichloromethane at low temperatures .
Key identifiers include:
- SMILES: BrC1=CC=C2C=3C1=CC=CC3CC2
- InChIKey: QALKJGMGKYKMKE-UHFFFAOYSA-N
- Synonyms: 5-Bromo-1,2-dihydroacenaphthylene, NSC 59823 .
Mechanism of Action
Target of Action
This compound is a heterocyclic compound with a unique structure of a fused five-membered ring and two six-membered rings. It is an important building block for many organic synthesis processes.
Mode of Action
It is known to be widely used in the synthesis of various organic compounds.
Biochemical Pathways
It has been used in the synthesis of various hetero- and carbocyclic compounds and complexes.
Result of Action
It is known to be an important building block in the synthesis of various organic compounds.
Biochemical Analysis
Biochemical Properties
1,2-Acenaphthylenedione, 5-bromo- plays a significant role in biochemical reactions, particularly in oxidative processes. It interacts with enzymes such as cytochrome P450, which is involved in the metabolism of various substrates. The compound can also interact with proteins that have active sites capable of accommodating its structure, leading to potential inhibition or activation of these proteins. Additionally, 1,2-Acenaphthylenedione, 5-bromo- can form adducts with nucleophiles, affecting the function of biomolecules it interacts with .
Cellular Effects
1,2-Acenaphthylenedione, 5-bromo- has been shown to influence various cellular processes. It can affect cell signaling pathways by interacting with key signaling molecules, leading to alterations in gene expression. This compound can also impact cellular metabolism by inhibiting or activating enzymes involved in metabolic pathways. Studies have demonstrated that 1,2-Acenaphthylenedione, 5-bromo- can induce oxidative stress in cells, leading to changes in cellular function and viability .
Molecular Mechanism
The molecular mechanism of 1,2-Acenaphthylenedione, 5-bromo- involves its ability to bind to specific biomolecules, leading to changes in their activity. This compound can inhibit enzymes by binding to their active sites, preventing substrate access. It can also activate certain proteins by inducing conformational changes. Additionally, 1,2-Acenaphthylenedione, 5-bromo- can modulate gene expression by interacting with transcription factors and other regulatory proteins .
Temporal Effects in Laboratory Settings
In laboratory settings, the effects of 1,2-Acenaphthylenedione, 5-bromo- can change over time. The compound’s stability and degradation are important factors that influence its long-term effects on cellular function. Studies have shown that 1,2-Acenaphthylenedione, 5-bromo- can degrade under certain conditions, leading to the formation of reactive intermediates that can further impact cellular processes. Long-term exposure to this compound can result in sustained oxidative stress and alterations in cellular homeostasis .
Dosage Effects in Animal Models
The effects of 1,2-Acenaphthylenedione, 5-bromo- vary with different dosages in animal models. At low doses, the compound may have minimal impact on cellular function, while higher doses can lead to significant changes in cellular metabolism and viability. Threshold effects have been observed, where a certain dosage level is required to elicit a measurable response. High doses of 1,2-Acenaphthylenedione, 5-bromo- can also result in toxic or adverse effects, including tissue damage and organ dysfunction .
Metabolic Pathways
1,2-Acenaphthylenedione, 5-bromo- is involved in various metabolic pathways, interacting with enzymes such as cytochrome P450 and other oxidoreductases. These interactions can lead to the formation of metabolites that may have different biological activities compared to the parent compound. The compound can also affect metabolic flux, altering the levels of key metabolites and influencing overall cellular metabolism .
Transport and Distribution
Within cells and tissues, 1,2-Acenaphthylenedione, 5-bromo- is transported and distributed through interactions with transporters and binding proteins. These interactions can affect the compound’s localization and accumulation in specific cellular compartments. The distribution of 1,2-Acenaphthylenedione, 5-bromo- can influence its biological activity and the extent of its effects on cellular function .
Biological Activity
1,2-Acenaphthylenedione, 5-bromo- (CAS No. 26254-35-3), also known as 5-Bromoacenaphthoquinone, is an organic compound with significant biological activity. This compound is a derivative of acenaphthoquinone and features a bromine atom at the 5th position of the acenaphthylene core. Its molecular formula is C12H5BrO2, and it has a molecular weight of approximately 261.07 g/mol. The compound is recognized for its interactions with various biological systems, particularly through its effects on enzyme activity and cellular processes.
1,2-Acenaphthylenedione, 5-bromo- exhibits notable biochemical properties that influence its biological activity:
- Enzyme Interactions : The compound interacts with cytochrome P450 enzymes and other oxidoreductases, which play crucial roles in drug metabolism and the biotransformation of various substrates. This interaction can lead to the formation of reactive metabolites that may have different biological effects compared to the parent compound.
- Formation of Adducts : It can form adducts with nucleophiles, affecting the function of biomolecules it interacts with. Such interactions may lead to inhibition or activation of specific proteins.
Cellular Effects
1,2-Acenaphthylenedione, 5-bromo- has been shown to influence several cellular processes:
- Cell Signaling Pathways : The compound can alter gene expression by interacting with key signaling molecules. This modulation can impact various cellular functions, including proliferation and apoptosis.
- Oxidative Stress Induction : Studies indicate that this compound can induce oxidative stress in cells, leading to changes in cell viability and function. Prolonged exposure may result in cellular damage and dysfunction.
Molecular Mechanism
The molecular mechanism underlying the biological activity of 1,2-Acenaphthylenedione, 5-bromo- involves:
- Binding to Biomolecules : The compound binds to specific biomolecules, leading to changes in their activity. This includes inhibition of enzymes by occupying their active sites and potentially activating other proteins through conformational changes.
- Modulation of Gene Expression : By interacting with transcription factors and regulatory proteins, this compound can influence gene expression patterns within cells.
Dosage Effects in Animal Models
Research on dosage effects reveals:
- At low doses, 1,2-Acenaphthylenedione, 5-bromo- may have minimal impact on cellular functions.
- Higher doses can lead to significant alterations in cellular metabolism and viability. Toxic effects have been observed at elevated dosages, including tissue damage and organ dysfunction.
Metabolic Pathways
The compound is involved in various metabolic pathways:
- Interaction with Metabolic Enzymes : It influences enzymes involved in metabolic flux, altering levels of key metabolites within cells. This interaction can have downstream effects on overall cellular metabolism.
Transport and Distribution
Transport mechanisms for 1,2-Acenaphthylenedione, 5-bromo- include:
- Cellular Transport : The compound's distribution within cells is facilitated by specific transporters and binding proteins. This localization is crucial for its biological activity and efficacy.
Study on Oxidative Stress Induction
A laboratory study demonstrated that exposure to varying concentrations of 1,2-Acenaphthylenedione, 5-bromo- resulted in increased levels of reactive oxygen species (ROS) in cultured human cell lines. The findings suggested a dose-dependent relationship where higher concentrations led to greater oxidative stress markers.
Neuroprotective Activity Investigation
In another study focusing on neuroprotective properties related to similar compounds, it was found that derivatives like 1,2-Acenaphthylenedione could potentially antagonize neurodegenerative effects associated with dopaminergic neuron degeneration. This suggests possible therapeutic avenues for neurodegenerative diseases.
Q & A
Q. Basic: What are the most effective synthetic routes for 5-bromo-1,2-acenaphthylenedione, and how do their yields compare?
Answer:
Two primary methods are documented:
- Sodium Borohydride Reduction : Reduction of 5-bromoacenaphthenequinone with NaBH₄ in isopropanol yields trans-5-bromoacenaphthene-1,2-diol with 57% post-recrystallization yield. This method avoids explosive reagents like LiAlH₄ and improves stereoselectivity .
- Bromoacetyl Bromide Route : Reacting acenaphthene with bromoacetyl bromide and AlCl₃ in CH₂Cl₂ yields 5-bromoacetylacenaphthene at 41.5% yield. This method requires careful temperature control (-20°C to RT) and purification via column chromatography .
Table 1: Comparison of Synthetic Routes
Method | Reagents | Yield | Key Advantage |
---|---|---|---|
NaBH₄ Reduction | NaBH₄, isopropanol | 57% | High stereoselectivity, safer |
Bromoacetyl Bromide | AlCl₃, CH₂Cl₂ | 41.5% | Direct functionalization |
Q. Basic: What safety precautions are critical when handling 5-bromo-1,2-acenaphthylenedione?
Answer:
- Hazards : Classified as causing skin/eye irritation (H315, H319) and respiratory irritation (H335) .
- PPE : Use nitrile gloves, chemical-resistant lab coats, and safety goggles. For aerosol-prone steps, employ P95 respirators (US) or EN 143-compliant masks (EU) .
- Ventilation : Use fume hoods to avoid inhalation of dust or vapors. Avoid dust formation during synthesis or purification .
Q. Basic: How do the physical properties of 5-bromo-1,2-acenaphthylenedione influence its experimental handling?
Answer:
- Melting Point : 257–259°C (decomposition), requiring high-temperature stability in reactions .
- Solubility : Insoluble in water but soluble in alcohols (e.g., ethanol, isopropanol), guiding solvent selection for recrystallization .
- Stability : Stable under dry, room-temperature storage but decomposes upon prolonged exposure to moisture or light .
Q. Advanced: How can stereoselectivity challenges in synthesizing trans-5-bromoacenaphthene-1,2-diol be mitigated?
Answer:
Traditional hydrolysis of halogenated precursors (e.g., 1,2,5-tribromoacenaphthene) yields predominantly cis-diols (25–26% yield) due to steric hindrance . To favor trans-isomers:
Reduction Optimization : Use NaBH₄ instead of LiAlH₄ for selective reduction of the diketone without over-reduction.
Chromatographic Separation : Separate cis/trans mixtures using silica gel chromatography with ethyl acetate/hexane gradients .
Q. Advanced: What analytical techniques are recommended for characterizing 5-bromo-1,2-acenaphthylenedione derivatives?
Answer:
- ¹H-NMR : Assigns stereochemistry (e.g., trans-diols show distinct coupling constants) and confirms bromine substitution patterns .
- Melting Point Analysis : Differentiates isomers (e.g., trans-diols have higher melting points than cis-diols) .
- Mass Spectrometry (MS) : Verifies molecular weight and bromine isotopic patterns.
Q. Advanced: What mechanistic insights explain the low yields in bromination reactions of acenaphthenequinone?
Answer:
- Electrophilic Aromatic Substitution (EAS) : Bromine preferentially attacks the electron-rich C5 position, but steric hindrance from adjacent substituents reduces reactivity.
- Side Reactions : Competing oxidation or dimerization under acidic/basic conditions further lowers yields. Mitigate via:
- Low-temperature reactions (-20°C) to suppress side pathways .
- Use of Lewis acids (e.g., AlCl₃) to enhance regioselectivity .
Q. Advanced: How can computational modeling predict the reactivity of 5-bromo-1,2-acenaphthylenedione in novel reactions?
Answer:
- DFT Calculations : Model electron density maps to identify nucleophilic/electrophilic sites. For example, predict bromine’s directing effects in EAS.
- Molecular Dynamics (MD) : Simulate solvent interactions to optimize reaction conditions (e.g., polarity effects in alcohol solvents) .
Q. Advanced: What gaps exist in ecotoxicological data for 5-bromo-1,2-acenaphthylenedione, and how might they be addressed?
Answer:
- Data Gaps : No PBT (persistence, bioaccumulation, toxicity) or biodegradability studies are available .
- Proposed Studies :
- Microbial Degradation Assays : Use soil microcosms to assess half-life.
- Aquatic Toxicity Testing : Evaluate LC₅₀ in Daphnia magna or zebrafish models.
- QSAR Modeling : Predict toxicity endpoints using quantitative structure-activity relationships.
Comparison with Similar Compounds
Comparison with Structurally Similar Compounds
5-Bromoacetylacenaphthene
- Synthesis : Prepared via Friedel-Crafts acylation of acenaphthene with bromoacetyl bromide, yielding an off-white solid (41.5% yield) .
- Spectroscopic Data :
- ¹H-NMR (CDCl₃) : δ 8.69 (d, J=8.4 Hz, aromatic H), 4.58 (s, CH₂).
- ¹³C-NMR : Aromatic carbons resonate between δ 120–140 ppm.
- Applications : Intermediate in synthesizing spiro compounds for medicinal chemistry .
Spiro[acenaphthylene-1,2'-pyrrolidin]-2-ones
- Examples :
- 4f : 3'-(4-Bromobenzoyl)-4',5'-diphenyl-2H-spiro compound.
- Melting Point : 176–178°C.
- HRMS : Calculated [M+H]⁺ 524.1024; Observed 524.1026 .
- Synthesis: Multi-component reactions involving acenaphthenequinone, aryl amines, and ketones under reflux conditions .
Acenaphthenequinone (1,2-Acenaphthylenedione)
- Structure : The diketone derivative of acenaphthene.
- Biological Relevance : Produced via bacterial oxidation of acenaphthene by Beijerinckia spp. .
- Comparison: Unlike 5-bromoacenaphthene, acenaphthenequinone lacks halogen substituents but shares applications in synthesizing spiro compounds and pharmaceuticals .
Physicochemical and Functional Comparisons
Physical Properties
Compound | Melting Point (°C) | Molecular Weight | Key Substituent |
---|---|---|---|
5-Bromoacenaphthene | 52 | 233.10 | Br |
5-Bromoacetylacenaphthene | Not reported | 301.16 | Br, COCH₂Br |
Spiro Compound 4f | 176–178 | 524.10 | Br, Ph, CO |
Acenaphthenequinone | 121–123 | 182.18 | O=C-C=O |
Key Research Findings
- Synthetic Efficiency : Brominated acenaphthene derivatives are synthesized via electrophilic substitution, but steric hindrance from the fused ring system limits reaction rates .
- Spectroscopic Trends: Bromine substituents deshield adjacent protons, shifting aromatic ¹H-NMR signals upfield (e.g., δ 8.69 in 5-bromoacetylacenaphthene vs. δ 7.3–8.1 in non-brominated analogs) .
- Thermal Stability : Brominated spiro compounds (e.g., 4f) exhibit higher melting points than chloro/fluoro analogs due to increased molecular symmetry and halogen size .
Preparation Methods
Oxidation of 5-Bromoacenaphthene
The most widely reported method involves the oxidation of 5-bromoacenaphthene to 1,2-acenaphthylenedione, 5-bromo- under acidic conditions. Sodium dichromate (Na₂Cr₂O₇·2H₂O) and cerium(III) chloride heptahydrate (CeCl₃·7H₂O) serve as the oxidizing agent and catalyst, respectively, in acetic acid.
Reaction Conditions
Procedure :
- Oxidation : 5-Bromoacenaphthene is stirred with Na₂Cr₂O₇·2H₂O and CeCl₃·7H₂O in acetic acid at 40°C for 8 hours.
- Workup : The mixture is quenched with cold water, filtered, and treated with aqueous sodium carbonate to remove unreacted oxidizing agents.
- Purification : The product is extracted with sodium bisulfate (4% aqueous solution), acidified with H₂SO₄, and recrystallized from ethanol to yield a yellow solid (mp > 238°C).
Spectral Characterization
The product is characterized by its distinct spectral signatures:
Technique | Key Peaks/Shifts | Source |
---|---|---|
¹H NMR | δ 8.37 (d, J = 8.2 Hz), 8.24–8.09 (m, 2H), 8.04 (d, J = 7.6 Hz), 7.93 (d, J = 7.2 Hz) in DMSO-d₆ | |
IR | 1730 cm⁻¹ (C=O stretching), 775 cm⁻¹ (C-Br) |
These data confirm the formation of the quinone structure with retained bromine substitution at the 5-position.
Alternative Synthetic Routes
Electrochemical Cleavage
Acenaphthenequinones can also be synthesized via electrochemical oxidation of acenaphthene derivatives. For example, oxidation of 5-bromoacenaphthene in the presence of oxygen may yield the quinone, though this method is less documented for mono-brominated derivatives.
Grignard Reactions
While Grignard reagents (e.g., 5-lithio-acenaphthene) have been used in coupling reactions with ketones, these routes primarily generate alkylation products rather than the quinone structure.
Reaction Mechanism
The oxidation of 5-bromoacenaphthene proceeds via epoxidation-dehydration or direct dehydrogenation , depending on the oxidizing agent. Sodium dichromate in acetic acid facilitates the cleavage of the central double bond, forming two ketone groups. Cerium(III) chloride may act as a Lewis acid to stabilize intermediates and enhance reaction efficiency.
Applications in Heterocyclic Synthesis
1,2-Acenaphthylenedione, 5-bromo- serves as a precursor for fused heterocycles. For instance, condensation with 3-amino-2-methyl-6-nitro-4(3H)-quinazolinone at 220°C yields brominated pyridazinoquinazoline derivatives, which are further functionalized into azo dyes.
Challenges and Optimization
- Selectivity : Competing side reactions (e.g., over-oxidation) necessitate precise control of temperature and stoichiometry.
- Catalyst Cost : Cerium(III) chloride adds to production expenses; alternative catalysts (e.g., manganese acetate) may require further evaluation.
Properties
IUPAC Name |
5-bromoacenaphthylene-1,2-dione | |
---|---|---|
Source | PubChem | |
URL | https://pubchem.ncbi.nlm.nih.gov | |
Description | Data deposited in or computed by PubChem | |
InChI |
InChI=1S/C12H5BrO2/c13-9-5-4-8-10-6(9)2-1-3-7(10)11(14)12(8)15/h1-5H | |
Source | PubChem | |
URL | https://pubchem.ncbi.nlm.nih.gov | |
Description | Data deposited in or computed by PubChem | |
InChI Key |
PHPQHHFMRUWUJG-UHFFFAOYSA-N | |
Source | PubChem | |
URL | https://pubchem.ncbi.nlm.nih.gov | |
Description | Data deposited in or computed by PubChem | |
Canonical SMILES |
C1=CC2=C(C=CC3=C2C(=C1)C(=O)C3=O)Br | |
Source | PubChem | |
URL | https://pubchem.ncbi.nlm.nih.gov | |
Description | Data deposited in or computed by PubChem | |
Molecular Formula |
C12H5BrO2 | |
Source | PubChem | |
URL | https://pubchem.ncbi.nlm.nih.gov | |
Description | Data deposited in or computed by PubChem | |
DSSTOX Substance ID |
DTXSID10457682 | |
Record name | 1,2-Acenaphthylenedione, 5-bromo- | |
Source | EPA DSSTox | |
URL | https://comptox.epa.gov/dashboard/DTXSID10457682 | |
Description | DSSTox provides a high quality public chemistry resource for supporting improved predictive toxicology. | |
Molecular Weight |
261.07 g/mol | |
Source | PubChem | |
URL | https://pubchem.ncbi.nlm.nih.gov | |
Description | Data deposited in or computed by PubChem | |
CAS No. |
26254-35-3 | |
Record name | 1,2-Acenaphthylenedione, 5-bromo- | |
Source | EPA DSSTox | |
URL | https://comptox.epa.gov/dashboard/DTXSID10457682 | |
Description | DSSTox provides a high quality public chemistry resource for supporting improved predictive toxicology. | |
Synthesis routes and methods
Procedure details
Disclaimer and Information on In-Vitro Research Products
Please be aware that all articles and product information presented on BenchChem are intended solely for informational purposes. The products available for purchase on BenchChem are specifically designed for in-vitro studies, which are conducted outside of living organisms. In-vitro studies, derived from the Latin term "in glass," involve experiments performed in controlled laboratory settings using cells or tissues. It is important to note that these products are not categorized as medicines or drugs, and they have not received approval from the FDA for the prevention, treatment, or cure of any medical condition, ailment, or disease. We must emphasize that any form of bodily introduction of these products into humans or animals is strictly prohibited by law. It is essential to adhere to these guidelines to ensure compliance with legal and ethical standards in research and experimentation.