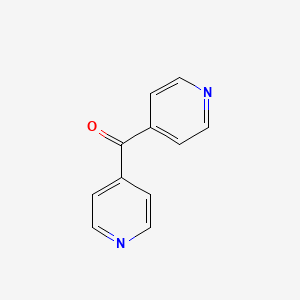
Di(pyridin-4-yl)methanone
Overview
Description
Di(pyridin-4-yl)methanone: is an organic compound with the molecular formula C₁₁H₈N₂O bis(4-pyridyl) ketone . This compound features two pyridine rings connected by a central carbonyl group, making it a versatile building block in organic synthesis and coordination chemistry.
Mechanism of Action
Target of Action
Di(pyridin-4-yl)methanone is primarily targeted towards protein kinases . Protein kinases are enzymes that modify other proteins by chemically adding phosphate groups to them (phosphorylation). This process is crucial in transmitting signals within cells (signal transduction) and regulating cellular activities such as cell division.
Mode of Action
The compound interacts with its protein kinase targets by inhibiting their activity . The planarity of the pyrido[3,4-g]quinazoline tricyclic system, which is a part of the compound’s structure, is essential for maintaining the protein kinase inhibitory potency . This suggests that the compound may bind to the active site of the protein kinase, preventing it from phosphorylating other proteins.
Pharmacokinetics
It has a molecular weight of 184.19400 , a density of 1.196g/cm3 , and a boiling point of 346.381ºC at 760 mmHg . These properties may influence the compound’s Absorption, Distribution, Metabolism, and Excretion (ADME) properties, and thus its bioavailability.
Result of Action
The primary result of this compound’s action is the inhibition of protein kinase activity . This can lead to a disruption of cellular signaling pathways, potentially altering cellular functions such as cell division and signal transduction. The specific molecular and cellular effects would depend on the particular protein kinases that the compound inhibits.
Action Environment
The action of this compound can be influenced by various environmental factors. For instance, the compound’s stability could be affected by temperature, as it has a melting point of 142°C (lit.) . Furthermore, the compound’s efficacy could be influenced by factors such as pH and the presence of other molecules in the cellular environment.
Biochemical Analysis
Biochemical Properties
Di(pyridin-4-yl)methanone plays a crucial role in biochemical reactions, particularly in the inhibition of protein kinases. It interacts with enzymes such as Cdc-like kinase 1 (CLK1) and Dual-specificity tyrosine-regulated kinase 1A (DYRK1A). These interactions are primarily due to the planar structure of the compound, which allows it to fit into the active sites of these enzymes, thereby inhibiting their activity . The compound’s ability to inhibit these kinases makes it a valuable tool in studying cell cycle regulation and signal transduction pathways.
Cellular Effects
This compound has been shown to affect various types of cells and cellular processes. It influences cell function by modulating cell signaling pathways, gene expression, and cellular metabolism. For instance, the inhibition of CLK1 and DYRK1A by this compound can lead to alterations in the phosphorylation status of key regulatory proteins, thereby affecting cell cycle progression and apoptosis . Additionally, this compound has been observed to impact cellular metabolism by altering the activity of metabolic enzymes, leading to changes in metabolite levels and energy production.
Molecular Mechanism
The molecular mechanism of this compound involves its binding interactions with biomolecules. The compound exerts its effects by fitting into the active sites of target enzymes, such as CLK1 and DYRK1A, and inhibiting their activity. This inhibition is achieved through the formation of hydrogen bonds and hydrophobic interactions between the compound and the enzyme’s active site residues . Furthermore, this compound can influence gene expression by modulating the activity of transcription factors and other regulatory proteins.
Temporal Effects in Laboratory Settings
In laboratory settings, the effects of this compound have been observed to change over time. The compound’s stability and degradation are critical factors that influence its long-term effects on cellular function. Studies have shown that this compound is relatively stable under standard laboratory conditions, but its activity can decrease over extended periods due to gradual degradation . Long-term exposure to the compound has been associated with sustained inhibition of target enzymes and prolonged alterations in cell signaling pathways and gene expression.
Dosage Effects in Animal Models
The effects of this compound vary with different dosages in animal models. At low doses, the compound effectively inhibits target enzymes without causing significant toxicity. At higher doses, this compound can exhibit toxic effects, including hepatotoxicity and nephrotoxicity . Threshold effects have been observed, where the compound’s efficacy plateaus beyond a certain dosage, indicating that optimal dosing is crucial for maximizing its therapeutic potential while minimizing adverse effects.
Metabolic Pathways
This compound is involved in several metabolic pathways, interacting with enzymes and cofactors that regulate its metabolism. The compound is primarily metabolized by cytochrome P450 enzymes, which catalyze its oxidation and subsequent conjugation with glucuronic acid or sulfate . These metabolic processes influence the compound’s bioavailability and clearance from the body, affecting its overall efficacy and safety profile.
Transport and Distribution
Within cells and tissues, this compound is transported and distributed through interactions with specific transporters and binding proteins. The compound can be actively transported across cell membranes by ATP-binding cassette (ABC) transporters, which facilitate its uptake and efflux . Additionally, binding proteins such as albumin can sequester this compound in the bloodstream, influencing its distribution and accumulation in target tissues.
Subcellular Localization
The subcellular localization of this compound is critical for its activity and function. The compound is known to localize in the cytoplasm and nucleus, where it interacts with target enzymes and regulatory proteins . Post-translational modifications, such as phosphorylation, can influence the compound’s localization by directing it to specific cellular compartments or organelles. These targeting signals ensure that this compound exerts its effects at the appropriate sites within the cell.
Preparation Methods
Synthetic Routes and Reaction Conditions: Di(pyridin-4-yl)methanone can be synthesized through various methods. One common approach involves the reaction of 4-bromopyridine with lithium diisopropylamide (LDA) , followed by the addition of carbon dioxide to form the corresponding carboxylic acid. This intermediate is then converted to the ketone using thionyl chloride and dimethylformamide (DMF) .
Industrial Production Methods: Industrial production of this compound typically involves the use of 4-cyanopyridine as a starting material. The nitrile group is hydrolyzed to the corresponding carboxylic acid, which is then subjected to Friedel-Crafts acylation using aluminum chloride and acetyl chloride to yield the desired ketone .
Chemical Reactions Analysis
Types of Reactions: Di(pyridin-4-yl)methanone undergoes various chemical reactions, including:
Oxidation: It can be oxidized to form the corresponding using oxidizing agents like .
Reduction: The compound can be reduced to the corresponding using reducing agents such as .
Substitution: It can participate in nucleophilic substitution reactions, where the pyridine rings can be functionalized with different substituents.
Common Reagents and Conditions:
Oxidation: Potassium permanganate in an acidic medium.
Reduction: Sodium borohydride in methanol.
Substitution: Various nucleophiles under basic conditions.
Major Products Formed:
Oxidation: Pyridine-4-carboxylic acid.
Reduction: Pyridin-4-ylmethanol.
Substitution: Substituted pyridine derivatives.
Scientific Research Applications
Di(pyridin-4-yl)methanone has a wide range of applications in scientific research:
Comparison with Similar Compounds
Di(2-pyridyl) ketone: Similar structure but with pyridine rings at the 2-position.
4,4’-Dipyridyl: Lacks the central carbonyl group, making it less versatile in certain reactions.
2-Benzoylpyridine: Features a benzene ring instead of a second pyridine ring.
Uniqueness: Di(pyridin-4-yl)methanone is unique due to its ability to form stable coordination complexes with various metal ions, making it highly valuable in coordination chemistry and materials science. Its dual pyridine rings also provide multiple sites for functionalization, enhancing its versatility in synthetic applications .
Properties
IUPAC Name |
dipyridin-4-ylmethanone | |
---|---|---|
Source | PubChem | |
URL | https://pubchem.ncbi.nlm.nih.gov | |
Description | Data deposited in or computed by PubChem | |
InChI |
InChI=1S/C11H8N2O/c14-11(9-1-5-12-6-2-9)10-3-7-13-8-4-10/h1-8H | |
Source | PubChem | |
URL | https://pubchem.ncbi.nlm.nih.gov | |
Description | Data deposited in or computed by PubChem | |
InChI Key |
CLAOXGJAXHTMPM-UHFFFAOYSA-N | |
Source | PubChem | |
URL | https://pubchem.ncbi.nlm.nih.gov | |
Description | Data deposited in or computed by PubChem | |
Canonical SMILES |
C1=CN=CC=C1C(=O)C2=CC=NC=C2 | |
Source | PubChem | |
URL | https://pubchem.ncbi.nlm.nih.gov | |
Description | Data deposited in or computed by PubChem | |
Molecular Formula |
C11H8N2O | |
Source | PubChem | |
URL | https://pubchem.ncbi.nlm.nih.gov | |
Description | Data deposited in or computed by PubChem | |
DSSTOX Substance ID |
DTXSID20484767 | |
Record name | Dipyridin-4-ylmethanone | |
Source | EPA DSSTox | |
URL | https://comptox.epa.gov/dashboard/DTXSID20484767 | |
Description | DSSTox provides a high quality public chemistry resource for supporting improved predictive toxicology. | |
Molecular Weight |
184.19 g/mol | |
Source | PubChem | |
URL | https://pubchem.ncbi.nlm.nih.gov | |
Description | Data deposited in or computed by PubChem | |
CAS No. |
6918-15-6 | |
Record name | Dipyridin-4-ylmethanone | |
Source | EPA DSSTox | |
URL | https://comptox.epa.gov/dashboard/DTXSID20484767 | |
Description | DSSTox provides a high quality public chemistry resource for supporting improved predictive toxicology. | |
Synthesis routes and methods
Procedure details
Retrosynthesis Analysis
AI-Powered Synthesis Planning: Our tool employs the Template_relevance Pistachio, Template_relevance Bkms_metabolic, Template_relevance Pistachio_ringbreaker, Template_relevance Reaxys, Template_relevance Reaxys_biocatalysis model, leveraging a vast database of chemical reactions to predict feasible synthetic routes.
One-Step Synthesis Focus: Specifically designed for one-step synthesis, it provides concise and direct routes for your target compounds, streamlining the synthesis process.
Accurate Predictions: Utilizing the extensive PISTACHIO, BKMS_METABOLIC, PISTACHIO_RINGBREAKER, REAXYS, REAXYS_BIOCATALYSIS database, our tool offers high-accuracy predictions, reflecting the latest in chemical research and data.
Strategy Settings
Precursor scoring | Relevance Heuristic |
---|---|
Min. plausibility | 0.01 |
Model | Template_relevance |
Template Set | Pistachio/Bkms_metabolic/Pistachio_ringbreaker/Reaxys/Reaxys_biocatalysis |
Top-N result to add to graph | 6 |
Feasible Synthetic Routes
Disclaimer and Information on In-Vitro Research Products
Please be aware that all articles and product information presented on BenchChem are intended solely for informational purposes. The products available for purchase on BenchChem are specifically designed for in-vitro studies, which are conducted outside of living organisms. In-vitro studies, derived from the Latin term "in glass," involve experiments performed in controlled laboratory settings using cells or tissues. It is important to note that these products are not categorized as medicines or drugs, and they have not received approval from the FDA for the prevention, treatment, or cure of any medical condition, ailment, or disease. We must emphasize that any form of bodily introduction of these products into humans or animals is strictly prohibited by law. It is essential to adhere to these guidelines to ensure compliance with legal and ethical standards in research and experimentation.