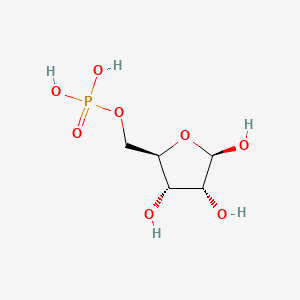
5-O-Phosphono-Beta-D-Ribofuranose
- Click on QUICK INQUIRY to receive a quote from our team of experts.
- With the quality product at a COMPETITIVE price, you can focus more on your research.
Overview
Description
Beta-D-Ribose-5-phosphate: is an organic compound that belongs to the class of pentose phosphates. It is a carbohydrate derivative containing a pentose substituted by one or more phosphate groups. This compound plays a crucial role in the pentose phosphate pathway, which is essential for nucleotide synthesis and cellular metabolism .
Preparation Methods
Synthetic Routes and Reaction Conditions: Beta-D-Ribose-5-phosphate can be synthesized through various routes. One common method involves the isomerization of ribulose-5-phosphate. This reaction is catalyzed by the enzyme ribose-5-phosphate isomerase . Another method involves the aldol condensation of glyceraldehyde-3-phosphate and dihydroxyacetone phosphate, followed by phosphorylation .
Industrial Production Methods: In industrial settings, beta-D-Ribose-5-phosphate is often produced using biotechnological methods. Microbial fermentation processes are employed, where genetically engineered microorganisms are used to convert glucose into ribose-5-phosphate. This method is preferred due to its efficiency and cost-effectiveness .
Chemical Reactions Analysis
Types of Reactions: Beta-D-Ribose-5-phosphate undergoes various chemical reactions, including:
Oxidation: It can be oxidized to form ribulose-5-phosphate.
Reduction: It can be reduced to form ribitol-5-phosphate.
Substitution: It can undergo substitution reactions to form different phosphorylated sugars.
Common Reagents and Conditions:
Oxidation: Common reagents include NADP+ and specific dehydrogenases.
Reduction: NADPH and specific reductases are used.
Substitution: Phosphorylating agents such as ATP are commonly used.
Major Products:
Oxidation: Ribulose-5-phosphate.
Reduction: Ribitol-5-phosphate.
Substitution: Various phosphorylated sugars.
Scientific Research Applications
Beta-D-Ribose-5-phosphate has numerous applications in scientific research:
Chemistry: It is used as a precursor in the synthesis of nucleotides and nucleic acids.
Biology: It plays a vital role in the pentose phosphate pathway, which is crucial for cellular metabolism and the production of NADPH.
Medicine: It is studied for its potential role in cancer metabolism and as a biomarker for certain diseases.
Industry: It is used in the production of riboflavin (vitamin B2) and other essential biomolecules.
Mechanism of Action
Beta-D-Ribose-5-phosphate exerts its effects primarily through its role in the pentose phosphate pathway. It is involved in the production of NADPH and ribose-5-phosphate, which are essential for nucleotide synthesis and cellular redox balance. The compound interacts with various enzymes, including ribose-5-phosphate isomerase and transketolase, to facilitate these processes .
Comparison with Similar Compounds
Ribulose-5-phosphate: An isomer of beta-D-Ribose-5-phosphate, involved in the same metabolic pathway.
Xylulose-5-phosphate: Another pentose phosphate involved in the pentose phosphate pathway.
Ribose-1-phosphate: A phosphorylated sugar involved in nucleotide metabolism.
Uniqueness: Beta-D-Ribose-5-phosphate is unique due to its specific role in the pentose phosphate pathway and its involvement in both oxidative and non-oxidative phases of the pathway. Its ability to act as a precursor for nucleotide synthesis and its role in maintaining cellular redox balance make it distinct from other similar compounds .
Properties
CAS No. |
34980-66-0 |
---|---|
Molecular Formula |
C5H11O8P |
Molecular Weight |
230.11 g/mol |
IUPAC Name |
[(2R,3S,4R,5R)-3,4,5-trihydroxyoxolan-2-yl]methyl dihydrogen phosphate |
InChI |
InChI=1S/C5H11O8P/c6-3-2(1-12-14(9,10)11)13-5(8)4(3)7/h2-8H,1H2,(H2,9,10,11)/t2-,3-,4-,5-/m1/s1 |
InChI Key |
KTVPXOYAKDPRHY-TXICZTDVSA-N |
Isomeric SMILES |
C([C@@H]1[C@H]([C@H]([C@@H](O1)O)O)O)OP(=O)(O)O |
Canonical SMILES |
C(C1C(C(C(O1)O)O)O)OP(=O)(O)O |
Origin of Product |
United States |
Disclaimer and Information on In-Vitro Research Products
Please be aware that all articles and product information presented on BenchChem are intended solely for informational purposes. The products available for purchase on BenchChem are specifically designed for in-vitro studies, which are conducted outside of living organisms. In-vitro studies, derived from the Latin term "in glass," involve experiments performed in controlled laboratory settings using cells or tissues. It is important to note that these products are not categorized as medicines or drugs, and they have not received approval from the FDA for the prevention, treatment, or cure of any medical condition, ailment, or disease. We must emphasize that any form of bodily introduction of these products into humans or animals is strictly prohibited by law. It is essential to adhere to these guidelines to ensure compliance with legal and ethical standards in research and experimentation.