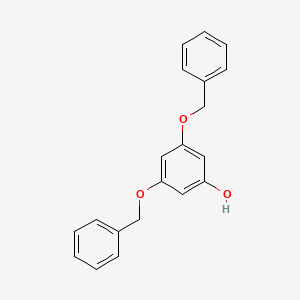
3,5-Dibenzyloxyphenol
Overview
Description
3,5-Dibenzyloxyphenol is a synthetic organic compound that falls under the category of disubstituted phenols. The compound is characterized by the presence of two benzyloxy groups attached to the phenol moiety at the 3 and 5 positions. This structure is a common motif in various chemical syntheses and can serve as an intermediate in the production of more complex molecules.
Synthesis Analysis
The synthesis of disubstituted phenols, such as 3,5-Dibenzyloxyphenol, can be achieved through robust and scalable methods. One such method involves the selective displacement of a halogen by nucleophilic aromatic substitution using a preformed mixture of potassium tert-butoxide and p-methoxybenzyl alcohol, followed by deprotection of the PMB ether with acid in the presence of 1,3-dimethoxybenzene . Although the specific synthesis of 3,5-Dibenzyloxyphenol is not detailed in the provided papers, the methodologies described can be adapted for its production.
Molecular Structure Analysis
The molecular structure of 3,5-Dibenzyloxyphenol would consist of a central benzene ring with two benzyloxy groups (-OCH2C6H5) at the 3 and 5 positions. The presence of these substituents can influence the electronic properties of the phenol ring and affect its reactivity. The molecular structure can be confirmed and analyzed using techniques such as NMR, IR, and elemental analysis, as demonstrated in the synthesis of related compounds .
Chemical Reactions Analysis
3,5-Dibenzyloxyphenol can undergo various chemical reactions typical of phenolic compounds. These reactions include further substitution reactions, oxidation, and the formation of metal complexes. For instance, phenolic compounds can participate in condensation reactions to form Schiff bases, as seen in the synthesis of complex organic structures involving phenolic moieties . Additionally, the hydroxyl groups in phenols can coordinate with metal ions to form metal complexes, which can exhibit interesting properties and potential applications, such as anticancer activity .
Physical and Chemical Properties Analysis
The physical and chemical properties of 3,5-Dibenzyloxyphenol would be influenced by the presence of the benzyloxy substituents. These groups are bulky and hydrophobic, which could affect the solubility of the compound in various solvents. The phenolic hydroxyl groups contribute to the potential for hydrogen bonding and the acidity of the phenol. The electronic properties, such as HOMO and LUMO energies, can be calculated using computational methods to predict reactivity and molecular properties . The compound's crystallinity and melting point can be determined through experimental methods, and its purity can be assessed by spectroscopic techniques .
Scientific Research Applications
Antioxidant and Catalytic Properties
- Mechanisms of Antioxidant Action: Research has demonstrated the antioxidant activity of sulphur-containing phenols, including structures similar to 3,5-Dibenzyloxyphenol. This activity is attributed to the generation of a Lewis acid catalyst, which can destroy a significant number of hydroperoxide molecules per mole of the sulphur compound. Such compounds, including 3,5-Dibenzyloxyphenol derivatives, are not initially antioxidants but may develop antioxidant properties through specific chemical transformations (Farzaliev, Fernando, & Scott, 1978).
Environmental Fate and Biotransformation
- Biotransformation Under Anaerobic Conditions: The environmental fate of chloroaromatic compounds, which are structurally related to 3,5-Dibenzyloxyphenol, has been studied under anaerobic conditions. These studies show how such compounds undergo biotransformation, which can lead to the formation of various byproducts, including bisphenolic compounds. This research is crucial for understanding the environmental impact and behavior of compounds like 3,5-Dibenzyloxyphenol (Verhagen, Swarts, Wijnberg, & Field, 1998).
Applications in Analytical Chemistry
- Chromogenic Systems for Assaying Uric Acid: 3,5-Dibenzyloxyphenol derivatives have been used in developing chromogenic detection systems for the enzymatic assay of uric acid in biological fluids. This demonstrates the potential use of 3,5-Dibenzyloxyphenol in the field of analytical chemistry, particularly in clinical diagnostics (Fossati & Prencipe, 2010).
Synthesis and Functionalization
- Synthesis of Phenolic Polymers: Recent research has focused on synthesizing linear phenolic polymers with controlled antioxidant, hydrophobicity, and surface adsorption properties. These polymers, which include functional groups similar to 3,5-Dibenzyloxyphenol, exhibit significant antiradical activity, making them suitable for various applications, including coatings (Hlushko, Hlushko, & Sukhishvili, 2018).
Potential in Drug Synthesis
- Intermediate for Synthesis of Flavonoids: The compound 3,5-Dibenzyloxyphenol has been used as an intermediate in the synthesis of bis-C-glucosyl flavonoids, demonstrating its potential application in the pharmaceutical industry for the synthesis of complex natural products (Kumazawa, Ishida, Matsuba, Sato, & Onodera, 1997).
properties
IUPAC Name |
3,5-bis(phenylmethoxy)phenol | |
---|---|---|
Source | PubChem | |
URL | https://pubchem.ncbi.nlm.nih.gov | |
Description | Data deposited in or computed by PubChem | |
InChI |
InChI=1S/C20H18O3/c21-18-11-19(22-14-16-7-3-1-4-8-16)13-20(12-18)23-15-17-9-5-2-6-10-17/h1-13,21H,14-15H2 | |
Source | PubChem | |
URL | https://pubchem.ncbi.nlm.nih.gov | |
Description | Data deposited in or computed by PubChem | |
InChI Key |
FEQJBICMACXNHS-UHFFFAOYSA-N | |
Source | PubChem | |
URL | https://pubchem.ncbi.nlm.nih.gov | |
Description | Data deposited in or computed by PubChem | |
Canonical SMILES |
C1=CC=C(C=C1)COC2=CC(=CC(=C2)O)OCC3=CC=CC=C3 | |
Source | PubChem | |
URL | https://pubchem.ncbi.nlm.nih.gov | |
Description | Data deposited in or computed by PubChem | |
Molecular Formula |
C20H18O3 | |
Source | PubChem | |
URL | https://pubchem.ncbi.nlm.nih.gov | |
Description | Data deposited in or computed by PubChem | |
DSSTOX Substance ID |
DTXSID70473868 | |
Record name | 3,5-dibenzyloxyphenol | |
Source | EPA DSSTox | |
URL | https://comptox.epa.gov/dashboard/DTXSID70473868 | |
Description | DSSTox provides a high quality public chemistry resource for supporting improved predictive toxicology. | |
Molecular Weight |
306.4 g/mol | |
Source | PubChem | |
URL | https://pubchem.ncbi.nlm.nih.gov | |
Description | Data deposited in or computed by PubChem | |
Product Name |
3,5-Dibenzyloxyphenol | |
CAS RN |
63604-98-8 | |
Record name | 3,5-dibenzyloxyphenol | |
Source | EPA DSSTox | |
URL | https://comptox.epa.gov/dashboard/DTXSID70473868 | |
Description | DSSTox provides a high quality public chemistry resource for supporting improved predictive toxicology. | |
Synthesis routes and methods
Procedure details
Retrosynthesis Analysis
AI-Powered Synthesis Planning: Our tool employs the Template_relevance Pistachio, Template_relevance Bkms_metabolic, Template_relevance Pistachio_ringbreaker, Template_relevance Reaxys, Template_relevance Reaxys_biocatalysis model, leveraging a vast database of chemical reactions to predict feasible synthetic routes.
One-Step Synthesis Focus: Specifically designed for one-step synthesis, it provides concise and direct routes for your target compounds, streamlining the synthesis process.
Accurate Predictions: Utilizing the extensive PISTACHIO, BKMS_METABOLIC, PISTACHIO_RINGBREAKER, REAXYS, REAXYS_BIOCATALYSIS database, our tool offers high-accuracy predictions, reflecting the latest in chemical research and data.
Strategy Settings
Precursor scoring | Relevance Heuristic |
---|---|
Min. plausibility | 0.01 |
Model | Template_relevance |
Template Set | Pistachio/Bkms_metabolic/Pistachio_ringbreaker/Reaxys/Reaxys_biocatalysis |
Top-N result to add to graph | 6 |
Feasible Synthetic Routes
Disclaimer and Information on In-Vitro Research Products
Please be aware that all articles and product information presented on BenchChem are intended solely for informational purposes. The products available for purchase on BenchChem are specifically designed for in-vitro studies, which are conducted outside of living organisms. In-vitro studies, derived from the Latin term "in glass," involve experiments performed in controlled laboratory settings using cells or tissues. It is important to note that these products are not categorized as medicines or drugs, and they have not received approval from the FDA for the prevention, treatment, or cure of any medical condition, ailment, or disease. We must emphasize that any form of bodily introduction of these products into humans or animals is strictly prohibited by law. It is essential to adhere to these guidelines to ensure compliance with legal and ethical standards in research and experimentation.