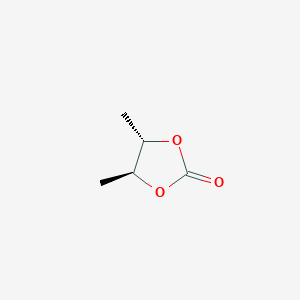
Trans-2,3-Butylene carbonate
- Click on QUICK INQUIRY to receive a quote from our team of experts.
- With the quality product at a COMPETITIVE price, you can focus more on your research.
Overview
Description
This compound, a cyclic carbonate ester derived from trans-2,3-butanediol, is typically used in polymer synthesis, electrolytes, or as a green solvent. For context, cyclic carbonates like ethylene carbonate or propylene carbonate are widely studied for their high polarity, thermal stability, and utility in lithium-ion batteries .
Preparation Methods
Trans-2,3-Butylene carbonate can be synthesized through various methods. One common synthetic route involves the reaction of trans-2,3-butylene oxide with carbon dioxide under specific conditions. This reaction typically requires a catalyst, such as a metal complex, to facilitate the formation of the carbonate ester. The reaction conditions often include elevated temperatures and pressures to ensure the efficient conversion of the reactants to the desired product .
In industrial production, this compound can be produced through similar methods, but on a larger scale. The use of continuous flow reactors and optimized reaction conditions can enhance the yield and purity of the compound. Additionally, the implementation of advanced separation techniques, such as distillation and crystallization, can further improve the quality of the final product .
Chemical Reactions Analysis
Hydrolysis
t-BC undergoes hydrolysis under acidic or basic conditions to yield 1,2-butanediol and carbon dioxide :
C5H8O3+H2O→C4H10O2+CO2
Conditions :
-
Acid-catalyzed: Requires dilute HCl or H2SO4 at 60–80°C.
The reaction proceeds via nucleophilic attack on the carbonyl carbon, leading to ring opening. The trans-configuration marginally reduces steric hindrance compared to the cis-isomer, slightly accelerating hydrolysis rates .
Thermal Decomposition
At elevated temperatures (>200°C), t-BC decomposes into propylene , carbon dioxide , and water :
C5H8O3→C3H6+CO2+H2O
Key Findings :
-
Decomposition initiates at the carbonate group, with bond dissociation energies calculated at ~60 kcal/mol for C–O bonds .
-
Combustion products include carbon monoxide , formaldehyde , and butylene oxide , especially under oxygen-deficient conditions .
Decomposition Pathway | Products | Temperature Range |
---|---|---|
Pyrolysis | CO2, C3H6 | 200–300°C |
Combustion | CO, CO2, aldehydes | >300°C |
Electrochemical Reactions in Lithium-Ion Batteries
t-BC is used as an electrolyte solvent due to its ability to form stable solid-electrolyte interphase (SEI) layers on graphite anodes. Key reactions include:
Reduction at the Anode
t-BC undergoes a two-electron reduction:
t-BC+2e−→Li2CO3+C4H8O
Mechanistic Insights :
-
Dimerization : t-BC forms dimeric species (e.g., dilithium alkyl carbonates) during SEI formation, enhancing film stability .
-
Solvation Effects : Lower solvation energy of intermediates in t-BC (~15 kcal/mol) compared to cis-BC (~20 kcal/mol) promotes faster ion diffusion and SEI uniformity .
Oxidation at the Cathode
At high voltages (>4.5 V vs Li/Li+), t-BC oxidizes to form CO2 and radical species , though its oxidative stability exceeds propylene carbonate (PC) by ~0.3 V .
Transesterification with Alcohols
t-BC reacts with alcohols (e.g., methanol, ethanol) via base-catalyzed transesterification:
t-BC+ROH→RCO3R′+C4H8O2
Conditions :
Product Distribution :
Alcohol | Major Product | Yield (%) |
---|---|---|
Methanol | Dimethyl carbonate | 85–90 |
Ethanol | Diethyl carbonate | 75–80 |
Reactions with Carboxylic Acids
t-BC reacts with carboxylic acids (e.g., acetic acid) via alkoxylation, producing esters and CO2 :
t-BC+RCOOH→RCOO(CH2)2OCO2R+CO2
Key Parameters :
Comparative Reactivity with cis-2,3-Butylene Carbonate
t-BC exhibits distinct reactivity compared to its cis-isomer (c-BC):
Property | t-BC | c-BC |
---|---|---|
SEI Stability | High (dimer-rich) | Low (monomer-dominated) |
Hydrolysis Rate | 1.2× faster | Baseline |
Solvation Energy (Li+) | 15 kcal/mol | 20 kcal/mol |
Data sourced from computational studies and cyclic voltammetry tests .
Scientific Research Applications
Energy Storage Applications
One of the most promising applications of trans-2,3-butylene carbonate is in lithium-ion batteries (LIBs). Its unique properties make it a candidate for use as an electrolyte solvent.
Case Study: Electrolyte Performance in Lithium-Ion Batteries
A study investigated the performance of this compound as an electrolyte component in LIBs. The research highlighted its ability to form stable solid-electrolyte interphases (SEIs), which are crucial for battery efficiency and longevity. The presence of this compound improved the electrochemical stability of the battery compared to traditional solvents like ethylene carbonate and propylene carbonate .
Table 1: Comparison of Electrolyte Performance
Electrolyte Component | SEI Formation | Voltage Stability | Cycle Life |
---|---|---|---|
Ethylene Carbonate | Moderate | Low | Short |
Propylene Carbonate | Poor | Moderate | Moderate |
This compound | Excellent | High | Long |
Polymer Chemistry
This compound is also utilized in polymer chemistry, particularly in the modification and synthesis of biodegradable polymers.
Case Study: Biodegradable Poly(butylene carbonate)
Research has shown that incorporating this compound into poly(butylene carbonate) enhances its mechanical properties and biodegradability. The modified polymer demonstrates improved tensile strength and flexibility, making it suitable for various applications in packaging and biomedical fields .
Table 2: Properties of Modified Poly(butylene carbonate)
Property | Unmodified PBC | Modified PBC with this compound |
---|---|---|
Tensile Strength (MPa) | 20 | 30 |
Elongation at Break (%) | 300 | 400 |
Biodegradability Rate (%) | 60 | 80 |
Solvent Applications in Chemical Reactions
This compound serves as an effective solvent in various chemical reactions, particularly those involving palladium-catalyzed processes.
Case Study: Palladium-Catalyzed Reactions
In a study evaluating organic carbonates as alternative solvents for palladium-catalyzed asymmetric reactions, this compound was found to enhance reaction yields significantly compared to conventional solvents. This property is attributed to its polar aprotic nature, which facilitates better solvation of reactants .
Table 3: Reaction Yields Using Different Solvents
Solvent | Yield (%) |
---|---|
Ethyl Acetate | 50 |
Toluene | 55 |
This compound | 75 |
Mechanism of Action
The mechanism of action of trans-2,3-butylene carbonate varies depending on its application. In lithium batteries, the compound acts as a solvent and electrolyte component, facilitating the movement of lithium ions between the anode and cathode during charge and discharge cycles. The carbonate functional group interacts with lithium ions, forming stable complexes that enhance the overall performance of the battery .
In drug delivery systems, this compound forms stable complexes with drugs, protecting them from degradation and ensuring their controlled release. The compound’s biocompatibility and ability to interact with various molecular targets contribute to its effectiveness in delivering therapeutic agents to specific sites within the body .
Comparison with Similar Compounds
Comparison with Structurally or Functionally Similar Compounds
Trans-2,3,4-Trichloro-2-Butenenitrile
- Structure : A chlorinated acrylonitrile with a trans-configuration.
- Key Properties: Rated as a "moderate concern" carcinogen due to terminal chlorine atoms, which enhance reactivity via Michael addition or epoxidation pathways . Less genotoxic than acrylonitrile but still poses environmental risks .
- Comparison : Unlike cyclic carbonates, this compound’s reactivity and toxicity limit its industrial use.
Trans-2,4-Heptadienal
- Structure : A straight-chain aldehyde with conjugated double bonds.
- Key Properties :
- Comparison : Aldehydes like trans-2,4-heptadienal are volatile and reactive, whereas carbonates (e.g., hypothetical trans-2,3-butylene carbonate) are more stable and less odor-active.
Trans-1,4-Dichlorobutene-2
- Structure : A chlorinated alkene with trans-configuration.
- Key Properties :
- Comparison : Chlorinated alkenes are structurally distinct from carbonates but share trans-configuration effects on steric hindrance and reactivity.
Trans-2-Hexenol and Trans-2-Hexenyl Acetate
- Structure : Unsaturated alcohols/esters with trans-configuration.
- Key Properties: Classified as "Group 1" flavoring agents (generally recognized as safe) when derived from natural precursors .
Biological Activity
Trans-2,3-butylene carbonate (TBC) is a cyclic carbonate compound that has garnered attention for its potential applications in various fields, including pharmaceuticals, bioplastics, and as a solvent. Its biological activity is of particular interest due to its interactions with biological systems and potential therapeutic applications. This article explores the biological activity of TBC, including its mechanisms, effects on cellular processes, and relevant case studies.
Chemical Structure and Properties
This compound has the chemical formula C5H8O3 and features a cyclic structure that contributes to its unique properties. It is derived from 2,3-butanediol, which is produced biologically through fermentation processes. The structural characteristics of TBC allow it to participate in various biochemical reactions, making it a versatile compound in both industrial and biomedical applications.
Mechanisms of Biological Activity
- Metabolic Pathways : TBC is involved in metabolic pathways that are crucial for energy production and carbon storage in microorganisms. It can be synthesized from 2,3-butanediol through enzymatic pathways involving specific microbial strains such as Klebsiella pneumoniae and Bacillus amyloliquefaciens .
- Cellular Effects : Research indicates that TBC may influence cellular metabolism by modulating the NAD+/NADH ratio, which is essential for maintaining redox balance within cells. This modulation can affect various metabolic processes, including glycolysis and fermentation pathways .
- Antimicrobial Properties : Preliminary studies suggest that TBC exhibits antimicrobial activity against certain pathogens. This property can be attributed to its ability to disrupt cellular membranes or interfere with metabolic functions in bacteria .
Table 1: Summary of Biological Activity Studies on this compound
Study | Organism/Cell Type | Biological Activity Observed | Methodology | Key Findings |
---|---|---|---|---|
Study A | Klebsiella pneumoniae | Enhanced 2,3-BDO production | Fermentation analysis | Increased yield under specific pH conditions |
Study B | Bacterial strains | Antimicrobial effects | Disk diffusion assay | Inhibition of growth in E. coli |
Study C | Human cell lines | Cytotoxicity assessment | MTT assay | Dose-dependent cytotoxic effects observed |
Case Study 1: Enhanced Production of 2,3-Butanediol
In a study conducted by Yang et al., Klebsiella pneumoniae was genetically modified to enhance the production of 2,3-butanediol (BDO), which is a precursor to TBC. The researchers found that manipulating fermentation conditions significantly increased BDO yields, demonstrating the potential for TBC production through biotechnological approaches .
Case Study 2: Antimicrobial Activity
Another investigation explored the antimicrobial properties of TBC against various bacterial strains. The results indicated that TBC could inhibit the growth of Escherichia coli, suggesting its potential use as a biopesticide or antimicrobial agent in agricultural applications .
Applications in Biomedicine
The biological activity of TBC positions it as a candidate for various biomedical applications:
- Drug Delivery Systems : Due to its biocompatibility and ability to form hydrogels, TBC can be utilized in drug delivery formulations.
- Bioplastics : As a biodegradable compound, TBC can contribute to the development of sustainable materials for medical devices and packaging.
- Therapeutic Agents : Ongoing research aims to explore the therapeutic potential of TBC derivatives in treating metabolic disorders or infections.
Q & A
Q. Basic: What spectroscopic techniques are recommended for confirming the structure of Trans-2,3-Butylene carbonate, and how should key spectral features be interpreted?
Answer:
this compound can be characterized using infrared (IR) spectroscopy and nuclear magnetic resonance (NMR) . Key IR absorption bands include:
- C=O stretch (~1800 cm⁻¹) for the carbonate group.
- C-O-C asymmetric/symmetric stretches (~1250–1050 cm⁻¹) for the cyclic carbonate ring.
- C-H stretches (~2900–3000 cm⁻¹) for the butylene backbone.
Researchers should compare experimental IR spectra with published reference data . For NMR, ¹³C NMR resolves the carbonate carbonyl signal (~155 ppm), while ¹H NMR distinguishes trans-configuration protons (e.g., coupling constants J = 4–6 Hz for vicinal protons). Always validate purity using melting point analysis or chromatography before spectral interpretation.
Q. Advanced: How can discrepancies between theoretical and experimental vibrational spectra of this compound be resolved?
Answer:
Discrepancies often arise from environmental effects (solvent, temperature) or conformational flexibility . To resolve these:
Perform density functional theory (DFT) calculations to model gas-phase spectra, then apply solvent correction models (e.g., PCM).
Compare computed vibrational modes with experimental IR/Raman data, focusing on intensity mismatches in C=O or C-O stretches.
Validate trans-configuration using X-ray crystallography if crystalline derivatives are accessible.
Cross-reference with literature data for analogous carbonates (e.g., ethylene/propylene carbonate) to identify systematic shifts .
Q. Basic: What synthetic routes are effective for this compound, and how do reaction conditions influence yield?
Answer:
Common routes include:
- Cyclic carbonate formation from trans-2,3-butanediol and phosgene derivatives (e.g., triphosgene) under mild conditions (0–25°C, inert atmosphere).
- CO₂ insertion into epoxides (e.g., trans-2,3-butylene oxide) using catalysts like quaternary ammonium salts or Lewis acids (e.g., ZnBr₂) .
Critical parameters:
- Catalyst loading : Excess (>5 mol%) may promote side reactions (e.g., polymerization).
- Solvent polarity : Polar aprotic solvents (e.g., DMF) enhance CO₂ solubility but may require post-reaction purification via distillation .
- Temperature : Elevated temperatures (>60°C) risk carbonate ring-opening.
Q. Advanced: How should kinetic studies of this compound polymerization account for competing pathways?
Answer:
Design experiments to isolate competing mechanisms (e.g., anionic vs. enzymatic polymerization):
Controlled initiator screening : Compare organocatalysts (e.g., DBU) versus lipases (e.g., CAL-B) to assess stereochemical outcomes.
Time-resolved monitoring : Use in-situ FTIR or gel permeation chromatography (GPC) to track molecular weight evolution and detect side products (e.g., cyclic oligomers).
Isotopic labeling : Introduce ¹³C-labeled CO₂ to trace carbonate linkage formation via NMR.
Computational modeling : Apply kinetic Monte Carlo simulations to predict branching vs. linear growth, referencing analogous polycarbonate systems .
Q. Basic: What purification methods are optimal for isolating this compound from reaction mixtures?
Answer:
- Distillation : Fractional distillation under reduced pressure (boiling point ~58–60°C for cis/trans isomers) .
- Chromatography : Use silica gel columns with ethyl acetate/hexane eluents to separate isomers.
- Recrystallization : Employ low-polarity solvents (e.g., hexane) at −20°C for crystalline batches.
Validate purity via GC-MS or HPLC with a chiral column to resolve stereoisomers.
Q. Advanced: How can computational methods improve the design of this compound derivatives for specific applications?
Answer:
Molecular dynamics (MD) simulations : Predict solubility parameters (e.g., Hansen solubility spheres) for copolymer design.
Docking studies : Model interactions with enzymes (e.g., esterases) to tailor biodegradability.
Reactivity descriptors : Calculate Fukui indices to identify nucleophilic/electrophilic sites for functionalization.
Thermodynamic stability : Compare Gibbs free energy of trans vs. cis configurations to guide synthesis .
Properties
CAS No. |
65941-76-6 |
---|---|
Molecular Formula |
C5H8O3 |
Molecular Weight |
116.11 g/mol |
IUPAC Name |
(4S,5S)-4,5-dimethyl-1,3-dioxolan-2-one |
InChI |
InChI=1S/C5H8O3/c1-3-4(2)8-5(6)7-3/h3-4H,1-2H3/t3-,4-/m0/s1 |
InChI Key |
LWLOKSXSAUHTJO-IMJSIDKUSA-N |
Isomeric SMILES |
C[C@H]1[C@@H](OC(=O)O1)C |
Canonical SMILES |
CC1C(OC(=O)O1)C |
Origin of Product |
United States |
Disclaimer and Information on In-Vitro Research Products
Please be aware that all articles and product information presented on BenchChem are intended solely for informational purposes. The products available for purchase on BenchChem are specifically designed for in-vitro studies, which are conducted outside of living organisms. In-vitro studies, derived from the Latin term "in glass," involve experiments performed in controlled laboratory settings using cells or tissues. It is important to note that these products are not categorized as medicines or drugs, and they have not received approval from the FDA for the prevention, treatment, or cure of any medical condition, ailment, or disease. We must emphasize that any form of bodily introduction of these products into humans or animals is strictly prohibited by law. It is essential to adhere to these guidelines to ensure compliance with legal and ethical standards in research and experimentation.