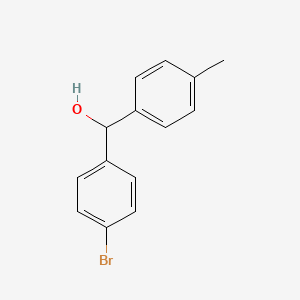
(4-Bromophenyl)(4-methylphenyl)methanol
Overview
Description
(4-Bromophenyl)(4-methylphenyl)methanol is an organic compound with the molecular formula C14H13BrO. It is a derivative of benzhydrol, where one phenyl group is substituted with a bromine atom at the para position and the other phenyl group is substituted with a methyl group at the para position.
Preparation Methods
Synthetic Routes and Reaction Conditions
The synthesis of (4-Bromophenyl)(4-methylphenyl)methanol typically involves the reaction of 4-bromobenzophenone with a suitable reducing agent. One common method is the reduction of 4-bromobenzophenone using sodium borohydride (NaBH4) in an alcoholic solvent such as methanol or ethanol. The reaction is carried out under mild conditions, typically at room temperature, to yield this compound .
Industrial Production Methods
In an industrial setting, the production of this compound may involve more scalable and efficient methods. One such method could be the catalytic hydrogenation of 4-bromobenzophenone using a palladium catalyst under hydrogen gas. This method allows for the selective reduction of the carbonyl group to a hydroxyl group while maintaining the integrity of the bromine and methyl substituents .
Chemical Reactions Analysis
Types of Reactions
(4-Bromophenyl)(4-methylphenyl)methanol can undergo various chemical reactions, including:
Oxidation: The hydroxyl group can be oxidized to a carbonyl group using oxidizing agents such as chromium trioxide (CrO3) or pyridinium chlorochromate (PCC).
Reduction: The compound can be further reduced to the corresponding hydrocarbon using strong reducing agents like lithium aluminum hydride (LiAlH4).
Common Reagents and Conditions
Oxidation: Chromium trioxide (CrO3) in acetic acid or pyridinium chlorochromate (PCC) in dichloromethane.
Reduction: Lithium aluminum hydride (LiAlH4) in dry ether or tetrahydrofuran (THF).
Substitution: Sodium methoxide (NaOCH3) in methanol or potassium tert-butoxide (KOtBu) in tert-butanol.
Major Products Formed
Oxidation: (4-Bromophenyl)(4-methylphenyl)ketone.
Reduction: (4-Bromophenyl)(4-methylphenyl)methane.
Substitution: Various substituted derivatives depending on the nucleophile used.
Scientific Research Applications
(4-Bromophenyl)(4-methylphenyl)methanol has several applications in scientific research:
Chemistry: It is used as an intermediate in the synthesis of more complex organic molecules, including pharmaceuticals and agrochemicals.
Biology: The compound can be used in the study of enzyme-catalyzed reactions involving hydroxyl and carbonyl groups.
Mechanism of Action
The mechanism of action of (4-Bromophenyl)(4-methylphenyl)methanol involves its interaction with various molecular targets and pathways. The hydroxyl group can participate in hydrogen bonding and other interactions with biological molecules, influencing their activity. The bromine and methyl substituents can also affect the compound’s reactivity and binding affinity to specific targets .
Comparison with Similar Compounds
Similar Compounds
(4-Bromophenyl)(phenyl)methanol: Similar structure but lacks the methyl group on the phenyl ring.
(4-Methylphenyl)(phenyl)methanol: Similar structure but lacks the bromine atom on the phenyl ring.
(4-Bromophenyl)(4-methylphenyl)ketone: Similar structure but with a carbonyl group instead of a hydroxyl group.
Uniqueness
The combination of these substituents provides a distinct set of properties that can be exploited in various chemical and industrial processes .
Properties
IUPAC Name |
(4-bromophenyl)-(4-methylphenyl)methanol | |
---|---|---|
Source | PubChem | |
URL | https://pubchem.ncbi.nlm.nih.gov | |
Description | Data deposited in or computed by PubChem | |
InChI |
InChI=1S/C14H13BrO/c1-10-2-4-11(5-3-10)14(16)12-6-8-13(15)9-7-12/h2-9,14,16H,1H3 | |
Source | PubChem | |
URL | https://pubchem.ncbi.nlm.nih.gov | |
Description | Data deposited in or computed by PubChem | |
InChI Key |
OZBSVWMYQPYYSC-UHFFFAOYSA-N | |
Source | PubChem | |
URL | https://pubchem.ncbi.nlm.nih.gov | |
Description | Data deposited in or computed by PubChem | |
Canonical SMILES |
CC1=CC=C(C=C1)C(C2=CC=C(C=C2)Br)O | |
Source | PubChem | |
URL | https://pubchem.ncbi.nlm.nih.gov | |
Description | Data deposited in or computed by PubChem | |
Molecular Formula |
C14H13BrO | |
Source | PubChem | |
URL | https://pubchem.ncbi.nlm.nih.gov | |
Description | Data deposited in or computed by PubChem | |
DSSTOX Substance ID |
DTXSID30471840 | |
Record name | (4-Bromophenyl)(4-methylphenyl)methanol | |
Source | EPA DSSTox | |
URL | https://comptox.epa.gov/dashboard/DTXSID30471840 | |
Description | DSSTox provides a high quality public chemistry resource for supporting improved predictive toxicology. | |
Molecular Weight |
277.16 g/mol | |
Source | PubChem | |
URL | https://pubchem.ncbi.nlm.nih.gov | |
Description | Data deposited in or computed by PubChem | |
CAS No. |
29334-17-6 | |
Record name | (4-Bromophenyl)(4-methylphenyl)methanol | |
Source | EPA DSSTox | |
URL | https://comptox.epa.gov/dashboard/DTXSID30471840 | |
Description | DSSTox provides a high quality public chemistry resource for supporting improved predictive toxicology. | |
Synthesis routes and methods I
Procedure details
Synthesis routes and methods II
Procedure details
Retrosynthesis Analysis
AI-Powered Synthesis Planning: Our tool employs the Template_relevance Pistachio, Template_relevance Bkms_metabolic, Template_relevance Pistachio_ringbreaker, Template_relevance Reaxys, Template_relevance Reaxys_biocatalysis model, leveraging a vast database of chemical reactions to predict feasible synthetic routes.
One-Step Synthesis Focus: Specifically designed for one-step synthesis, it provides concise and direct routes for your target compounds, streamlining the synthesis process.
Accurate Predictions: Utilizing the extensive PISTACHIO, BKMS_METABOLIC, PISTACHIO_RINGBREAKER, REAXYS, REAXYS_BIOCATALYSIS database, our tool offers high-accuracy predictions, reflecting the latest in chemical research and data.
Strategy Settings
Precursor scoring | Relevance Heuristic |
---|---|
Min. plausibility | 0.01 |
Model | Template_relevance |
Template Set | Pistachio/Bkms_metabolic/Pistachio_ringbreaker/Reaxys/Reaxys_biocatalysis |
Top-N result to add to graph | 6 |
Feasible Synthetic Routes
Disclaimer and Information on In-Vitro Research Products
Please be aware that all articles and product information presented on BenchChem are intended solely for informational purposes. The products available for purchase on BenchChem are specifically designed for in-vitro studies, which are conducted outside of living organisms. In-vitro studies, derived from the Latin term "in glass," involve experiments performed in controlled laboratory settings using cells or tissues. It is important to note that these products are not categorized as medicines or drugs, and they have not received approval from the FDA for the prevention, treatment, or cure of any medical condition, ailment, or disease. We must emphasize that any form of bodily introduction of these products into humans or animals is strictly prohibited by law. It is essential to adhere to these guidelines to ensure compliance with legal and ethical standards in research and experimentation.