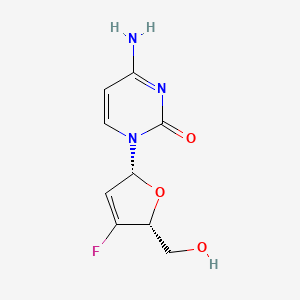
Cytidine, 2',3'-didehydro-2',3'-dideoxy-3'-fluoro-
- Click on QUICK INQUIRY to receive a quote from our team of experts.
- With the quality product at a COMPETITIVE price, you can focus more on your research.
Overview
Description
3’-Fluoro-2’,3’-dideoxydidehydrocytidine is a fluorinated nucleoside analog. It is structurally similar to natural nucleosides but contains a fluorine atom at the 3’ position and lacks hydroxyl groups at the 2’ and 3’ positions. This compound has garnered significant interest due to its potential antiviral properties, particularly against human immunodeficiency virus (HIV).
Preparation Methods
Synthetic Routes and Reaction Conditions
The synthesis of 3’-Fluoro-2’,3’-dideoxydidehydrocytidine typically involves multiple steps, starting from readily available precursorsThe key intermediate, 1-O-benzoyl-3E-fluoro-3,4-unsaturated-5,6-di(tert-butyldimethylsilyloxy)-2-hexanone, is obtained through a series of reactions including protection, fluorination, and deprotection steps .
Industrial Production Methods
Industrial production methods for this compound are designed to maximize yield and purity while minimizing costs and environmental impact. These methods often involve large-scale synthesis using optimized reaction conditions and purification techniques. The use of automated reactors and continuous flow systems can enhance the efficiency and scalability of the production process.
Chemical Reactions Analysis
Types of Reactions
3’-Fluoro-2’,3’-dideoxydidehydrocytidine undergoes various chemical reactions, including:
Oxidation: This reaction can introduce additional functional groups or modify existing ones.
Reduction: This reaction can convert double bonds to single bonds, altering the compound’s structure.
Substitution: This reaction involves the replacement of one functional group with another, often using nucleophilic or electrophilic reagents.
Common Reagents and Conditions
Common reagents used in these reactions include:
Oxidizing agents: Such as potassium permanganate or chromium trioxide.
Reducing agents: Such as lithium aluminum hydride or sodium borohydride.
Substitution reagents: Such as halogenating agents or organometallic compounds.
Major Products Formed
The major products formed from these reactions depend on the specific conditions and reagents used. For example, oxidation can yield ketones or aldehydes, while reduction can produce alcohols or alkanes. Substitution reactions can introduce various functional groups, such as halides or alkyl groups .
Scientific Research Applications
3’-Fluoro-2’,3’-dideoxydidehydrocytidine has a wide range of scientific research applications, including:
Chemistry: Used as a building block for the synthesis of more complex molecules.
Biology: Studied for its interactions with enzymes and nucleic acids.
Medicine: Investigated for its potential as an antiviral agent, particularly against HIV.
Industry: Utilized in the development of new pharmaceuticals and diagnostic tools.
Mechanism of Action
The mechanism of action of 3’-Fluoro-2’,3’-dideoxydidehydrocytidine involves its incorporation into viral DNA during replication. The fluorine atom at the 3’ position disrupts the normal function of the nucleoside, leading to chain termination and inhibition of viral replication. This compound targets viral reverse transcriptase, an enzyme critical for the replication of retroviruses like HIV .
Comparison with Similar Compounds
Similar Compounds
3’-Fluoro-2’,3’-dideoxyuridine: Another fluorinated nucleoside analog with antiviral properties.
3’-Fluoro-3’-deoxythymidine: Known for its potent inhibition of HIV replication.
2’,3’-Dideoxy-3’-fluorouridine: Studied for its potential use as an antiviral agent.
Uniqueness
3’-Fluoro-2’,3’-dideoxydidehydrocytidine is unique due to its specific structural modifications, which confer distinct antiviral properties. The presence of the fluorine atom at the 3’ position and the absence of hydroxyl groups at the 2’ and 3’ positions enhance its ability to inhibit viral replication while reducing toxicity compared to other nucleoside analogs .
Properties
CAS No. |
125362-05-2 |
---|---|
Molecular Formula |
C9H10FN3O3 |
Molecular Weight |
227.19 g/mol |
IUPAC Name |
4-amino-1-[(2R,5R)-4-fluoro-5-(hydroxymethyl)-2,5-dihydrofuran-2-yl]pyrimidin-2-one |
InChI |
InChI=1S/C9H10FN3O3/c10-5-3-8(16-6(5)4-14)13-2-1-7(11)12-9(13)15/h1-3,6,8,14H,4H2,(H2,11,12,15)/t6-,8-/m1/s1 |
InChI Key |
MEVXHGRLPDLNHY-HTRCEHHLSA-N |
Isomeric SMILES |
C1=CN(C(=O)N=C1N)[C@H]2C=C([C@H](O2)CO)F |
Canonical SMILES |
C1=CN(C(=O)N=C1N)C2C=C(C(O2)CO)F |
Origin of Product |
United States |
Disclaimer and Information on In-Vitro Research Products
Please be aware that all articles and product information presented on BenchChem are intended solely for informational purposes. The products available for purchase on BenchChem are specifically designed for in-vitro studies, which are conducted outside of living organisms. In-vitro studies, derived from the Latin term "in glass," involve experiments performed in controlled laboratory settings using cells or tissues. It is important to note that these products are not categorized as medicines or drugs, and they have not received approval from the FDA for the prevention, treatment, or cure of any medical condition, ailment, or disease. We must emphasize that any form of bodily introduction of these products into humans or animals is strictly prohibited by law. It is essential to adhere to these guidelines to ensure compliance with legal and ethical standards in research and experimentation.