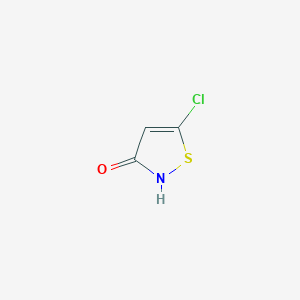
5-Chloroisothiazol-3-ol
- Click on QUICK INQUIRY to receive a quote from our team of experts.
- With the quality product at a COMPETITIVE price, you can focus more on your research.
Overview
Description
5-Chloroisothiazol-3-ol: is an organic compound with the molecular formula C3H2ClNOS . It is a member of the isothiazole family, characterized by a five-membered ring containing both sulfur and nitrogen atoms. This compound is known for its antimicrobial properties and is used in various industrial applications .
Preparation Methods
Synthetic Routes and Reaction Conditions: 5-Chloroisothiazol-3-ol can be synthesized through several methods. One common route involves the reaction of 3-hydroxyisothiazole with thionyl chloride, resulting in the chlorination of the isothiazole ring . Another method includes the reaction of 3-hydroxyisothiazole with phosphorus pentachloride under controlled conditions .
Industrial Production Methods: Industrial production of this compound typically involves large-scale chlorination processes. The reaction is carried out in a controlled environment to ensure high yield and purity. The product is then purified through recrystallization or distillation .
Chemical Reactions Analysis
Types of Reactions:
Oxidation: 5-Chloroisothiazol-3-ol can undergo oxidation reactions, leading to the formation of sulfoxides and sulfones.
Reduction: Reduction of this compound can yield various reduced forms, such as thiols.
Substitution: This compound can participate in substitution reactions, where the chlorine atom is replaced by other functional groups.
Common Reagents and Conditions:
Oxidation: Common oxidizing agents include hydrogen peroxide and potassium permanganate.
Reduction: Reducing agents such as lithium aluminum hydride and sodium borohydride are used.
Substitution: Nucleophiles like amines and thiols are commonly used in substitution reactions.
Major Products Formed:
Oxidation: Sulfoxides and sulfones.
Reduction: Thiols and other reduced forms.
Substitution: Various substituted isothiazoles.
Scientific Research Applications
Chemistry: 5-Chloroisothiazol-3-ol is used as a building block in the synthesis of more complex molecules. Its unique structure allows for the creation of diverse chemical compounds .
Biology: In biological research, this compound is studied for its antimicrobial properties. It is effective against a wide range of bacteria and fungi, making it valuable in the development of new antibiotics .
Medicine: This compound is explored for its potential therapeutic applications, including its use as an antimicrobial agent in medical formulations .
Industry: this compound is widely used as a biocide in industrial water treatment, paints, and coatings to prevent microbial growth and biofouling .
Mechanism of Action
The antimicrobial activity of 5-Chloroisothiazol-3-ol is attributed to its ability to inhibit life-sustaining enzymes, specifically those with thiol groups at their active sites. The compound forms mixed disulfides with these enzymes, disrupting their function and leading to microbial cell death .
Comparison with Similar Compounds
- Methylisothiazolinone
- Chloromethylisothiazolinone
- Benzisothiazolinone
- Octylisothiazolinone
- Dichlorooctylisothiazolinone
- Butylbenzisothiazolinone
Uniqueness: 5-Chloroisothiazol-3-ol stands out due to its specific chlorine substitution, which enhances its antimicrobial properties compared to other isothiazolones. This unique feature makes it particularly effective in industrial applications where strong biocidal activity is required .
Properties
IUPAC Name |
5-chloro-1,2-thiazol-3-one |
Source
|
---|---|---|
Source | PubChem | |
URL | https://pubchem.ncbi.nlm.nih.gov | |
Description | Data deposited in or computed by PubChem | |
InChI |
InChI=1S/C3H2ClNOS/c4-2-1-3(6)5-7-2/h1H,(H,5,6) |
Source
|
Source | PubChem | |
URL | https://pubchem.ncbi.nlm.nih.gov | |
Description | Data deposited in or computed by PubChem | |
InChI Key |
XFIPRDRBFGJGIZ-UHFFFAOYSA-N |
Source
|
Source | PubChem | |
URL | https://pubchem.ncbi.nlm.nih.gov | |
Description | Data deposited in or computed by PubChem | |
Canonical SMILES |
C1=C(SNC1=O)Cl |
Source
|
Source | PubChem | |
URL | https://pubchem.ncbi.nlm.nih.gov | |
Description | Data deposited in or computed by PubChem | |
Molecular Formula |
C3H2ClNOS |
Source
|
Source | PubChem | |
URL | https://pubchem.ncbi.nlm.nih.gov | |
Description | Data deposited in or computed by PubChem | |
DSSTOX Substance ID |
DTXSID10452011 |
Source
|
Record name | 5-chloroisothiazol-3-ol | |
Source | EPA DSSTox | |
URL | https://comptox.epa.gov/dashboard/DTXSID10452011 | |
Description | DSSTox provides a high quality public chemistry resource for supporting improved predictive toxicology. | |
Molecular Weight |
135.57 g/mol |
Source
|
Source | PubChem | |
URL | https://pubchem.ncbi.nlm.nih.gov | |
Description | Data deposited in or computed by PubChem | |
CAS No. |
25629-58-7 |
Source
|
Record name | 5-chloroisothiazol-3-ol | |
Source | EPA DSSTox | |
URL | https://comptox.epa.gov/dashboard/DTXSID10452011 | |
Description | DSSTox provides a high quality public chemistry resource for supporting improved predictive toxicology. | |
Disclaimer and Information on In-Vitro Research Products
Please be aware that all articles and product information presented on BenchChem are intended solely for informational purposes. The products available for purchase on BenchChem are specifically designed for in-vitro studies, which are conducted outside of living organisms. In-vitro studies, derived from the Latin term "in glass," involve experiments performed in controlled laboratory settings using cells or tissues. It is important to note that these products are not categorized as medicines or drugs, and they have not received approval from the FDA for the prevention, treatment, or cure of any medical condition, ailment, or disease. We must emphasize that any form of bodily introduction of these products into humans or animals is strictly prohibited by law. It is essential to adhere to these guidelines to ensure compliance with legal and ethical standards in research and experimentation.