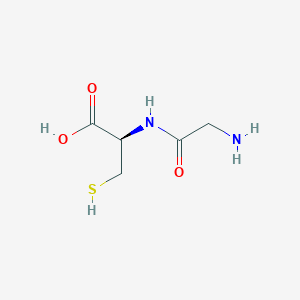
Glycylcysteine
Overview
Description
Glycylcysteine is a dipeptide composed of glycine and L-cysteine residues. It is a small molecule with the chemical formula C5H10N2O3S and a molecular weight of 178.21 g/mol . This compound is known for its role as a metabolite and its involvement in various biological processes .
Mechanism of Action
Target of Action
Glycylcysteine, a dipeptide formed from glycine and L-cysteine residues , is a metabolite . It is capable of accepting a hydron from a donor (Bronsted acid) . .
Mode of Action
It is known to have a bronsted base property, indicating that it can accept a hydron from a donor . This property may play a role in its interactions with its targets.
Biochemical Pathways
It is known to be a metabolite , suggesting that it may play a role in metabolic processes
Result of Action
As a metabolite , it may play a role in various metabolic processes
Biochemical Analysis
Biochemical Properties
Glycylcysteine is involved in several biochemical reactions, primarily due to its thiol group from cysteine, which can participate in redox reactions. It interacts with enzymes such as γ-glutamyl transpeptidase, which catalyzes the transfer of the γ-glutamyl group from glutathione to amino acids or peptides . This interaction is crucial for the metabolism of glutathione, an important antioxidant in cells. This compound also interacts with other biomolecules like proteins and peptides, forming disulfide bonds that stabilize protein structures.
Cellular Effects
This compound influences various cellular processes, including cell signaling pathways, gene expression, and cellular metabolism. It has been shown to protect cells from oxidative stress by enhancing the synthesis of glutathione . This protection is vital for maintaining cellular homeostasis and preventing damage from reactive oxygen species. Additionally, this compound can modulate cell signaling pathways involved in apoptosis and inflammation, thereby influencing cell survival and immune responses.
Molecular Mechanism
At the molecular level, this compound exerts its effects through several mechanisms. It can bind to enzymes and proteins, altering their activity. For instance, this compound can inhibit or activate enzymes involved in redox reactions, thereby influencing cellular redox states . It also affects gene expression by modulating transcription factors and other regulatory proteins. These interactions can lead to changes in the expression of genes involved in antioxidant defense, apoptosis, and other cellular processes.
Temporal Effects in Laboratory Settings
In laboratory settings, the effects of this compound can vary over time. Its stability and degradation are influenced by factors such as temperature and pH . This compound is relatively stable at neutral pH but can degrade under acidic or basic conditions. Over time, its protective effects on cells may diminish as it degrades, leading to reduced efficacy in long-term experiments. Its short-term effects, such as enhancing glutathione synthesis, are well-documented.
Dosage Effects in Animal Models
The effects of this compound vary with different dosages in animal models. At low doses, it can enhance antioxidant defenses and protect against oxidative stress . At high doses, it may exhibit toxic effects, such as inducing oxidative stress or disrupting cellular metabolism. The threshold for these effects depends on the specific animal model and experimental conditions. It is crucial to determine the optimal dosage to maximize its beneficial effects while minimizing potential toxicity.
Metabolic Pathways
This compound is involved in several metabolic pathways, including the synthesis and degradation of glutathione . It interacts with enzymes such as γ-glutamyl transpeptidase and glutathione synthetase, which are essential for glutathione metabolism. These interactions influence metabolic flux and the levels of metabolites involved in redox reactions and detoxification processes. This compound also participates in the transsulfuration pathway, contributing to the synthesis of cysteine and other sulfur-containing compounds.
Transport and Distribution
Within cells and tissues, this compound is transported and distributed through various mechanisms. It can be taken up by cells via amino acid transporters and distributed to different cellular compartments . This compound can also bind to proteins and peptides, influencing its localization and accumulation. These interactions are crucial for its role in cellular redox regulation and antioxidant defense.
Subcellular Localization
This compound is localized in various subcellular compartments, including the cytosol, mitochondria, and endoplasmic reticulum . Its subcellular localization is influenced by targeting signals and post-translational modifications. In the mitochondria, this compound plays a role in maintaining redox balance and protecting against oxidative damage. In the endoplasmic reticulum, it contributes to protein folding and quality control by forming disulfide bonds with nascent proteins.
Preparation Methods
Synthetic Routes and Reaction Conditions
Glycylcysteine can be synthesized through peptide coupling reactions. One common method involves the use of carbodiimide coupling agents, such as dicyclohexylcarbodiimide (DCC), to facilitate the formation of the peptide bond between glycine and L-cysteine . The reaction typically occurs in an organic solvent like dimethylformamide (DMF) under mild conditions.
Industrial Production Methods
Industrial production of this compound may involve solid-phase peptide synthesis (SPPS) techniques, which allow for the efficient and scalable production of peptides. In SPPS, the amino acids are sequentially added to a growing peptide chain anchored to a solid resin, followed by cleavage and purification steps .
Chemical Reactions Analysis
Types of Reactions
Glycylcysteine undergoes various chemical reactions, including:
Oxidation: The thiol group in L-cysteine can be oxidized to form disulfide bonds.
Reduction: Disulfide bonds can be reduced back to thiol groups.
Substitution: The amino and carboxyl groups can participate in substitution reactions.
Common Reagents and Conditions
Oxidation: Hydrogen peroxide (H2O2) or other oxidizing agents.
Reduction: Dithiothreitol (DTT) or other reducing agents.
Substitution: Carbodiimides for peptide bond formation.
Major Products Formed
Oxidation: Formation of cystine (disulfide-linked cysteine).
Reduction: Regeneration of free thiol groups.
Substitution: Formation of peptide bonds with other amino acids.
Scientific Research Applications
Glycylcysteine has a wide range of applications in scientific research:
Chemistry: Used as a building block for the synthesis of more complex peptides and proteins.
Biology: Studied for its role in cellular metabolism and as a precursor for glutathione synthesis.
Industry: Utilized in the production of pharmaceuticals and nutraceuticals.
Comparison with Similar Compounds
Similar Compounds
Cysteine: An amino acid with a thiol group, involved in protein synthesis and redox reactions.
Glutathione: A tripeptide composed of glutamate, cysteine, and glycine, known for its antioxidant properties.
N-Acetylcysteine: A derivative of cysteine used as a medication and supplement for its mucolytic and antioxidant effects.
Uniqueness of Glycylcysteine
This compound is unique due to its specific role as a dipeptide precursor for glutathione synthesis. Unlike cysteine, which is a single amino acid, this compound combines the properties of both glycine and L-cysteine, making it a valuable intermediate in biochemical pathways .
Biological Activity
Glycylcysteine (Gly-Cys) is a dipeptide composed of glycine and cysteine, with the molecular formula CHNOS and a molecular weight of 178.21 g/mol. This compound has garnered attention for its diverse biological activities, particularly in the fields of cellular metabolism, antioxidant defense, and potential therapeutic applications.
This compound plays a crucial role in various biochemical processes, primarily due to the thiol group from cysteine, which participates in redox reactions. Key properties include:
- Antioxidant Activity : this compound is a precursor for glutathione synthesis, an important antioxidant that protects cells from oxidative stress.
- Enzyme Interactions : It interacts with enzymes such as γ-glutamyl transpeptidase, facilitating the transfer of γ-glutamyl groups to amino acids or peptides.
The biological activity of this compound can be attributed to several mechanisms:
- Cell Signaling : It influences cellular signaling pathways and gene expression, enhancing cellular metabolism and antioxidant defenses.
- Redox Regulation : this compound can modulate the activity of various enzymes involved in redox reactions, impacting the cellular redox state.
- Subcellular Localization : It is distributed across various cellular compartments such as the cytosol and mitochondria, where it exerts its effects.
3.1 Antioxidant Properties
This compound has been shown to enhance glutathione levels in cells, providing protection against oxidative damage. A study indicated that at low doses, this compound significantly improved antioxidant defenses in animal models exposed to oxidative stress.
3.2 Inhibition of Thrombin Activatable Fibrinolysis Inhibitor (TAFI)
Research identified this compound as a potent inhibitor of TAFI, which plays a role in fibrinolysis. In vitro assays demonstrated that this compound exhibited an inhibitory constant (K(app)(i)) of 0.14 µM for TAFI, suggesting its potential use in therapies targeting thrombotic conditions .
4.1 Clinical Applications
This compound's biological activity has led to investigations in various clinical settings:
- Oxidative Stress Management : Clinical trials are exploring its efficacy in conditions characterized by oxidative stress, such as neurodegenerative diseases and cancer.
- Thrombosis Treatment : Its role as a TAFI inhibitor positions this compound as a candidate for developing new anticoagulant therapies .
5. Comparative Analysis
The following table summarizes key characteristics and findings related to this compound compared to other cysteine derivatives:
Compound | Molecular Weight | Antioxidant Activity | TAFI Inhibition | Clinical Application |
---|---|---|---|---|
This compound | 178.21 g/mol | Yes | Yes (K(app)(i)=0.14 µM) | Neurodegenerative diseases |
Guanidinyl-L-cysteine | 175.22 g/mol | Moderate | Yes (IC(50)=9.4 µM) | Potential anticoagulant therapy |
γ-Glutamyl-cysteine | 197.24 g/mol | Yes | No | Antioxidant supplementation |
6. Future Directions
Further research is warranted to explore:
- Bioavailability : Studies indicate low oral bioavailability (~1% in rats), necessitating alternative delivery methods for effective therapeutic use.
- Mechanistic Studies : More detailed investigations into the molecular mechanisms underlying its biological activities are needed to fully understand its potential applications.
Properties
IUPAC Name |
(2R)-2-[(2-aminoacetyl)amino]-3-sulfanylpropanoic acid | |
---|---|---|
Source | PubChem | |
URL | https://pubchem.ncbi.nlm.nih.gov | |
Description | Data deposited in or computed by PubChem | |
InChI |
InChI=1S/C5H10N2O3S/c6-1-4(8)7-3(2-11)5(9)10/h3,11H,1-2,6H2,(H,7,8)(H,9,10)/t3-/m0/s1 | |
Source | PubChem | |
URL | https://pubchem.ncbi.nlm.nih.gov | |
Description | Data deposited in or computed by PubChem | |
InChI Key |
MFBYPDKTAJXHNI-VKHMYHEASA-N | |
Source | PubChem | |
URL | https://pubchem.ncbi.nlm.nih.gov | |
Description | Data deposited in or computed by PubChem | |
Canonical SMILES |
C(C(C(=O)O)NC(=O)CN)S | |
Source | PubChem | |
URL | https://pubchem.ncbi.nlm.nih.gov | |
Description | Data deposited in or computed by PubChem | |
Isomeric SMILES |
C([C@@H](C(=O)O)NC(=O)CN)S | |
Source | PubChem | |
URL | https://pubchem.ncbi.nlm.nih.gov | |
Description | Data deposited in or computed by PubChem | |
Molecular Formula |
C5H10N2O3S | |
Source | PubChem | |
URL | https://pubchem.ncbi.nlm.nih.gov | |
Description | Data deposited in or computed by PubChem | |
DSSTOX Substance ID |
DTXSID30428632 | |
Record name | glycylcysteine | |
Source | EPA DSSTox | |
URL | https://comptox.epa.gov/dashboard/DTXSID30428632 | |
Description | DSSTox provides a high quality public chemistry resource for supporting improved predictive toxicology. | |
Molecular Weight |
178.21 g/mol | |
Source | PubChem | |
URL | https://pubchem.ncbi.nlm.nih.gov | |
Description | Data deposited in or computed by PubChem | |
CAS No. |
57281-78-4 | |
Record name | Glycyl-L-cysteine | |
Source | CAS Common Chemistry | |
URL | https://commonchemistry.cas.org/detail?cas_rn=57281-78-4 | |
Description | CAS Common Chemistry is an open community resource for accessing chemical information. Nearly 500,000 chemical substances from CAS REGISTRY cover areas of community interest, including common and frequently regulated chemicals, and those relevant to high school and undergraduate chemistry classes. This chemical information, curated by our expert scientists, is provided in alignment with our mission as a division of the American Chemical Society. | |
Explanation | The data from CAS Common Chemistry is provided under a CC-BY-NC 4.0 license, unless otherwise stated. | |
Record name | glycylcysteine | |
Source | EPA DSSTox | |
URL | https://comptox.epa.gov/dashboard/DTXSID30428632 | |
Description | DSSTox provides a high quality public chemistry resource for supporting improved predictive toxicology. | |
Retrosynthesis Analysis
AI-Powered Synthesis Planning: Our tool employs the Template_relevance Pistachio, Template_relevance Bkms_metabolic, Template_relevance Pistachio_ringbreaker, Template_relevance Reaxys, Template_relevance Reaxys_biocatalysis model, leveraging a vast database of chemical reactions to predict feasible synthetic routes.
One-Step Synthesis Focus: Specifically designed for one-step synthesis, it provides concise and direct routes for your target compounds, streamlining the synthesis process.
Accurate Predictions: Utilizing the extensive PISTACHIO, BKMS_METABOLIC, PISTACHIO_RINGBREAKER, REAXYS, REAXYS_BIOCATALYSIS database, our tool offers high-accuracy predictions, reflecting the latest in chemical research and data.
Strategy Settings
Precursor scoring | Relevance Heuristic |
---|---|
Min. plausibility | 0.01 |
Model | Template_relevance |
Template Set | Pistachio/Bkms_metabolic/Pistachio_ringbreaker/Reaxys/Reaxys_biocatalysis |
Top-N result to add to graph | 6 |
Feasible Synthetic Routes
Q1: What is the structural characterization of glycylcysteine?
A1: this compound is a dipeptide composed of glycine and cysteine linked by a peptide bond. Its molecular formula is C5H9NO3S, and its molecular weight is 163.19 g/mol. While specific spectroscopic data wasn't detailed in the provided research, peptides like this compound can be characterized using techniques like nuclear magnetic resonance (NMR) spectroscopy and mass spectrometry.
Q2: Are there alternative compounds with similar biological activities to this compound?
A: Yes, research suggests that other cysteine-containing compounds share similar biological activities with this compound. For instance, both allyl-cysteine and γ-glutamyl-cysteine-ethylester exhibited inhibitory effects on osteoclastogenesis in vitro, similar to this compound []. These findings point towards the importance of the cysteine moiety in the observed biological activity.
Q3: What are the limitations of using this compound in its pure form for oral applications?
A: One major limitation is the low bioavailability of orally administered this compound. A pilot study in rats revealed that the bioavailability of the compound in an aqueous solution was only about 1% []. This low bioavailability suggests that the compound is likely being degraded or poorly absorbed in the digestive tract, leading to limited systemic availability. Further research is needed to explore alternative delivery methods or formulations that could enhance the bioavailability of this compound.
Disclaimer and Information on In-Vitro Research Products
Please be aware that all articles and product information presented on BenchChem are intended solely for informational purposes. The products available for purchase on BenchChem are specifically designed for in-vitro studies, which are conducted outside of living organisms. In-vitro studies, derived from the Latin term "in glass," involve experiments performed in controlled laboratory settings using cells or tissues. It is important to note that these products are not categorized as medicines or drugs, and they have not received approval from the FDA for the prevention, treatment, or cure of any medical condition, ailment, or disease. We must emphasize that any form of bodily introduction of these products into humans or animals is strictly prohibited by law. It is essential to adhere to these guidelines to ensure compliance with legal and ethical standards in research and experimentation.